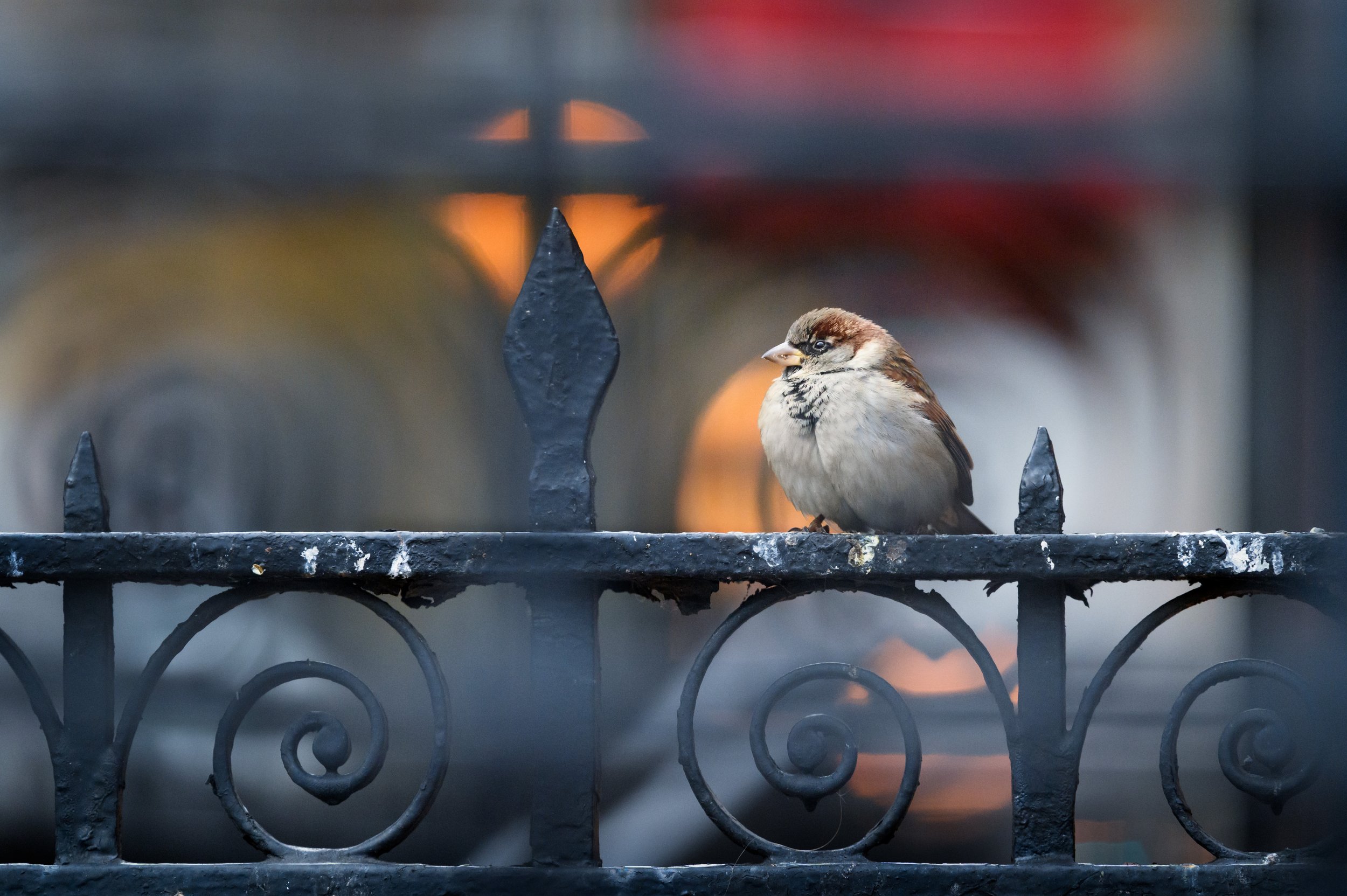
Library
Browse resources published by our research team.
In addition to full texts of our peer-reviewed articles, our library includes research digests that break down our peer-reviewed articles; in-depth reports that thoroughly examine a topic; commentaries that explain the significance of particular issues in wild animal welfare science; and short communications that briefly survey a field or topic.
Wild Animal Initiative adheres to Open Science TOP Guidelines. Read more here.
Wildlife contraception and welfare
Simon Eckerström Liedholm discusses why contraception is a promising tool for improving wild animal welfare, and what further research is most urgently needed.
Contraception as a promising tool for welfare improvements
When resources are scarce, wild animals sometimes die of starvation (Gordon et al. 1988), dehydration, or exposure due to a lack of suitable shelters (Sibly & Hone 2002, Hill et al. 2019), all of which cause significant suffering (Gregory 2008). In many species, the competition for resources is especially strong among juvenile animals (Sol et al. 1998, Ward et al. 2006). Naively, making more resources available seems like it would solve the resource scarcity problem, but increasing the amount of available resources will often lead to higher fertility rates and juvenile survival rates, which eventually leads to larger populations instead (Prevedello et al. 2013, Ruffino et al. 2014), such that the competition for resources does not decrease in the long term.
One simple approach to find out how many animals are negatively affected by resource scarcity is to measure the fraction of deaths that are directly attributed to starvation. However, such an approach plausibly underestimates the pervasiveness of resource scarcity, due to interactions among different types of causes of death. For example, predation appears to be the most common cause of death for several taxa and life stages (Hill et al. 2019, Collins & Kays 2011), but whilst it is the proximate or final cause when it happens, it may not always be the ultimate cause — the underlying reason the lethal event occurred. For example, an animal that is starving may be prone to taking risks (Anholt & Werner 1995), or too weak to run away, which causes them to be more exposed to predators, making them more likely to be eaten. Therefore, even for some proximate causes of death that do not seem to be related to resource scarcity, there could be an underlying scarcity-related risk factor. In that case, a reduction of one cause of mortality (e.g. predation) might be partially or fully ‘compensated’ by the increase of another cause of mortality (e.g. starvation) such that life expectancy doesn’t change, known as compensatory mortality (Bergman et al. 2015). If everyone who is starving is eaten by a predator just before they would otherwise die of starvation, it would superficially appear like starvation wasn’t a problem at all, if we base our judgment solely on the proximate cause of death (predation in this case). Nevertheless, resource scarcity does appear to be a fairly common phenomenon (Prevedello et al. 2013), and irrespective of the ultimate cause of death, living a life of near starvation is a negative experience and of welfare concern. However, since resource limitations often are restraining population sizes (Prevedello et al. 2013), many populations will simply grow in response to increased resource availability, resulting in a larger population with similar levels of starvation.
Contraception provides one mechanism by which resource scarcity could be reduced, without inducing a corresponding increase in population size (Hecht 2021). Moreover, contraception may improve individual well-being through the alleviation of resource scarcity in other ways as well. For example, contraception could improve the welfare of the offspring by reducing sibling competition for resources (Mendl 1988, Andersen et al. 2011, Hudson et al. 2011). It could also reduce the burden of gestation and childrearing for some parents by allowing them to save energy and invest more in maintaining body condition (Kirkwood 1977, Kirkwood & Rose 1991, Lemaître et al. 2015). The cost of parental care, and thus benefits of reducing it, are dependent on the species and sex of the individual (Goldberg et al. 2020, Santos & Nakagawa 2012), which will cause additional variation in the net welfare effect of contraception.
There are several studies in which the effects of contraceptives on proxies for welfare have been investigated in wild animals (see Supplementary Table 2 in the review by Gray & Cameron 2010), and they seem to show both negative and positive welfare effects. The negative effects that were detected for some species/methods are related to maladaptive social behavior and inflammation at injection sites, among other things (Gray & Cameron 2010). The plausibly positive effects that have been found are related to survival and body weight, which are the types of benefits that we would predict given the model outlined in the previous paragraph. Several of the studies on survival detected increases (see for instance: Twigg et al. 2001, Turner & Kirkpatrick 2002, Williams et al. 2007), but one of them found no effect (Saunders et al. 2002), and two of them observed mixed results (Ransom et al. 2013 and Bromley & Gese 2001). Many studies have looked for changes in body weight, and several of them have found increases in treated females (see Table S2 in Gray & Cameron 2010). Such increases in body weight could serve as a proxy for welfare through improved nutritional status, but it could also be a reflection of changes in satiety and the foraging risk-reward trade off, where the ultimate effect on welfare is less clear. Many studies have had very small sample sizes, lacked control groups, and focused on a narrow set of welfare relevant traits, so it is hard to draw any strong conclusions. However, it seems very likely that the type, target and delivery mechanism of contraceptives have contributed to the observed differences in welfare-related outcomes among studies.
It is important to note though, that wildlife contraceptives haven’t been developed or applied with the goal of improving wild animal welfare by alleviating resource scarcity. As an analogy: even though a squirt gun is ineffective at putting out large fires — it was never intended for that use — water delivered in the right way is nonetheless very useful when there is a fire. Similarly, it is perhaps no surprise that the currently available evidence on the welfare effects is inconclusive, if no one has intentionally tried to improve wild animal welfare with it. It is the compelling theoretical potential that wildlife contraception offers for significant welfare improvement for large numbers of individuals that makes us excited to see further research in this area.
A very brief history of wildlife contraception research
As of 2011 (Kirkpatrick et al. 2011), wildlife contraception had been developed and successfully tested in 85 species of animals (mostly mammals), and work on different forms of wildlife contraception have continued since then (Asa & Moresco 2019). There are several different forms of contraceptives available for wild animals. Vaccine-based contraceptives (so-called immunocontraceptives) like the porcine zona pellucida (PZP) vaccine and the gonadotropin-releasing hormone (GnRH) vaccine were developed for feral horses and other wild mammals in the 1980s, and are still being used today (Asa & Moresco 2019). Steroid hormonal contraception methods have also been investigated, although high costs, toxicity, and problems with delivery (Kirkpatrick et al. 2011) have caused these methods to mostly be abandoned. Surgical sterilization has also been used to reduce fertility in wild populations of animals (Denicola & Denicola 2021), but because of the invasiveness of the procedure, it is likely more detrimental to animal welfare than other methods (Hampton 2017). It is also oftentimes more expensive per treated animal (Kirkpatrick et al. 2011). More recently, contraceptives have been developed for birds (Nicarbazin), such as pigeons and geese, and 4-vinylcyclohexene diepoxide (VCD) developed for rats (See box). These contraceptives prevent fertilization (in the case of Nicarbazin) or development of germ cells (in the case of VCD), and have only recently become available as commercial products. Products like these open up the potential for large-scale application of wildlife contraceptives, given the abundance of pigeons and rats in the world, and the potential for use in other related species.
Future research
Although scientists have conducted wildlife contraception research for decades, the focus of this research has largely not been on the welfare impacts of contraception, most likely because the primary goal has been to reduce population sizes of pest or managed species, rather than to improve welfare. Consequently, more research is needed to address the many unanswered questions regarding the possible connection between contraception and welfare. Examples of such questions include: can we expect better or worse welfare outcomes in certain taxa? If there is strong interspecific competition, will contraception lead to compensatory population growth and resource use by competitor species, eliminating the potential positive effects?
In addition to ecological considerations of contraceptive use, each of the methods requires additional scrutiny. How do different delivery methods, such as oral baits, injections and surgical sterilizations differ in their welfare effects? What are the welfare related side-effects, and in what contexts do they outweigh the possible positive welfare benefits? What are the long-term welfare effects to the treated animals? What are the indirect effects from changes in population sizes on the welfare of individuals belonging to other species?
Finally, the inter-individual dynamics of different animal populations generate additional research questions. For example, do the effects of contraception on welfare differ between solitary and social species? One reason we might expect different welfare outcomes in social and solitary species is that for social species, fewer offspring could affect the collective gathering of resources or protection from outside threats. A smaller group could be suboptimal relative to a larger social group, and contraception could therefore reduce the group size below its optimum and have negative welfare implications. Conversely, individuals of solitary species might mostly experience competitive interactions with conspecifics, where a smaller population size would be more likely to have positive welfare effects through reduced competition.
We outline a few potential projects that could help answer some of the questions regarding the welfare aspects of wildlife contraception:
Project idea 1: Empirically test the welfare effects of a contraceptive agent, with a focus on both the direct and indirect effects on individuals within the population. See an example for such a project on pigeons. Another target could be rodents (using, for example, the product ContraPest) or other species that have high fertility (with potential to improve the welfare of many individuals).
Project idea 2: Empirically or theoretically test whether and how sociality interacts with the potential welfare effects of contraception.
Project idea 3: Empirically test whether different delivery methods have different average welfare outcomes, and whether it is possible to predict which method will suit different types of species.
Project idea 4: Empirically explore the factors that determine perceptions of wildlife contraceptives, and determine public support for making improvements beyond the ‘natural’ welfare-level as opposed to just minimizing harms.
For all of the possible projects mentioned above, studying both adults and juveniles would be highly valuable when possible, given that we expect somewhat different mechanisms to mediate the welfare effects in adults compared to the welfare effects on juveniles. For instance, we would expect any reduction in sibling competition to primarily affect juvenile welfare.
Box 1
When looking for ways to test hypotheses about the welfare effects of contraceptives on wild animals, we want to look for projects where there is a potential for improvement to a lot of individuals. Rats (Rattus spp.) seem highly promising in that regard, given that they have high fertility rates. The reproductive strategy of rats results in a large number of ‘excess’ individuals who will most likely die before they reproduce. By reducing competition, contraception might increase the fraction of juveniles surviving to reproductive maturity, even if there is no change in the size of the adult population. Humans are also already exploring contraceptive interventions for them. ContraPest (developed by SenesTech) is a type of contraceptive that can be used for rats and other rodents in the United States. Testing the welfare effects of ContraPest on rodents, or indeed trying to further cut costs or develop additional types of contraceptives for rodents, seem like very promising avenues for further research.
Pigeons (Columba livia) are another promising target species for studying the welfare effects of wildlife contraceptives, as they are also very common, and there is a commercially available contraceptive for them (OvoControl, produced by Innolytics). The fertility rate of pigeons is lower than the fertility rate of rats, which therefore likely affects the potential for juvenile welfare improvement. Nevertheless, the available contraceptive for pigeons appears to be somewhat cheaper than for rats, as is indicated by my rough cost estimate for applying OvoControl and ContraPest to populations of pigeons and rats, respectively. In addition, public support for pigeon welfare (and other bird species) improvements is likely to be greater than for rats (Jaeger & Wilks 2021), so studying the welfare effects of OvoControl might have greater potential for widespread implementation. Hence, studying the welfare effects of pigeon contraception might be the most promising option, in order to eventually achieve welfare improvements for wild animals through contraception.
While currently available data does not clearly demonstrate welfare improvements from contraceptive applications, there are good theoretical reasons to expect that contraception could be extremely effective under the right conditions. Therefore, if we explicitly try to use contraception to improve wild animal welfare, it has the potential to improve the living conditions of many wild animals, especially in populations where resource scarcity is pervasive. Wildlife contraception holds great potential to improve wild animal welfare, and research to address the key theoretical and empirical questions should be a priority.
Early-life experiences are a priority in wild animal welfare research
Hecht, L. (2021). The importance of considering age when quantifying wild animals’ welfare. Biological Reviews, 96(6), 2602-2616.
Luke Hecht explains why Wild Animal Initiative’s first call for grant proposals is focused on the welfare of juvenile animals.
Wild Animal Initiative recently announced our first public call for proposals, which focuses on the welfare and ecology of juvenile wild animals. Holding calls for proposals on specific themes allows us to showcase the pillars and breadth of wild animal welfare research. Here, I will explain why we think it is right to prioritize the interests of juvenile animals in most cases, drawing on my recent paper in Biological Reviews.
In many species, a majority of individuals die before reaching adulthood. For the typical wild animal, the welfare they could expect as an adult is irrelevant — but all animals experience at least some of what life is like as a juvenile. If our moral priority is making sure that as many animals as possible experience good welfare, then our practical priority should be ensuring the well-being of juvenile wild animals.
In my paper (and earlier blog post) on the importance of considering age when quantifying wild animals’ welfare, this thinking is formalized with the concept of “welfare expectancy.” Welfare expectancy represents the expected sum of welfare that a typical newborn individual will experience over their lifetime, accounting in theory for the distribution of possible welfare and lifespan outcomes. Depending on how welfare and survival rates vary with age in a given species, welfare expectancy may be especially responsive to changes in welfare and/or survival rate during particular periods of life (“welfare elasticity”, a form of proportional sensitivity analysis; Manlik et al. 2017).
If we assume there is no age-specific variation in survival rates or welfare, then lifetime welfare expectancy will always be most responsive to interventions benefiting the youngest individuals, since they will be the most numerous age group. In reality, survival rate and welfare likely do vary with age in nearly all species. It is often juveniles and senescent (very old) adults who are most vulnerable to disease, starvation and predation. Therefore, it is plausible that individuals in these demographics experience lower welfare on average than prime-age adults.
When might early-life experiences be less important to an animal’s expected lifetime welfare? In species with low juvenile survivorship and long adult life expectancies, the amount of time lived as an adult by the minority of individuals who survive their early years could exceed the amount of time lived by all the many individuals who died as juveniles. In this scenario, our priorities would likely be split between efforts to improve juvenile survival (so that a greater proportion of individuals reach adulthood) and efforts to improve adult welfare.
Species that meet these conditions tend to be less numerous, however, and are therefore unlikely to be among our priorities in the near future. For example, even North American black bears — potentially long-lived animals — appear to collectively live a majority (~55%) of their years as juveniles (based on baseline vital rates from Lewis et al. 2014). For each study or intervention, it will ultimately be important to define their target demographics and estimate how many animal life-years could potentially be improved in quality.
The everyday experiences of juvenile wild animals are little understood, yet could be highly impactful for wild animal welfare. We are excited to review your proposals for research on this important and neglected topic.
See here for more details.
Reducing the burden of disease: the One Health approach
Jane Capozzelli
Jane Capozzelli reviews the One Health approach to public health and veterinary medicine, identifying research themes that could be prioritized to most improve wild animal welfare.
Abstract
I review the One Health approach to public health and veterinary medicine and present three contemporary research projects.
One Health is motivated by the maxim that the health and well-being of domestic animals, people, and wildlife are inextricably linked. Many projects focus on mitigating wildlife diseases in ways that help humans or livestock.
These projects can benefit the welfare of wild animals by improving the target animals’ health. To maximize wild animal welfare benefits, One Health projects should prioritize targeting common species and particularly prolonged or painful health issues.
Key takeaways
Although One Health does not optimize for wild animal welfare, it represents a tractable opportunity to expand existing efforts in a beneficial direction.
One Health researchers seem to care about wildlife health for the sake of wild animals’ welfare, not just because of the ways wildlife health issues affect people.
Demonstrating that improving wild animal welfare can also benefit public health or livestock health might appeal to people who do not typically prioritize animal welfare.
Established approaches for wildlife health research are relatively easy to extend to high-priority issues in wild animal welfare.
Introduction to One Health
Health, though not the only determinant of good welfare, is a key component (Mellor and Beausoleil 2015). By replacing feeling sick with feeling healthy, good health improves welfare. For the purposes of this article, when I refer to improvements to wild animal welfare, I mainly refer to improving the health of target animals.
Wildlife health projects are promising avenues for improving wild animal welfare. Many of these projects are designed using the “One Health” approach. The framework views both the human and non-human worlds as morally important and interconnected (Schwabe 1984, Zinsstag et al. 2001). For example, veterinarians swear an oath to use their knowledge for both “the promotion of public health” and “the prevention and relief of animal suffering” (Mackenzie et al. 2013). More specifically, One Health follows the maxim that the health and welfare of animals and people are inextricably linked to each other and to the quality of the environment (Osborn et al. 2009).
One Health has been highly successful relative to other health paradigms (Osofsky and Pongsiri 2018). Historically, wildlife health, livestock health, and human health were treated as separate problems. But solving today’s most complex health problems needs expertise in all three areas (Whitmee et al. 2015). Addressing wildlife health, livestock health, and human health collectively — as part of “One Health” — ultimately yields benefits that can’t be found by addressing each problem individually.
Some leading institutions in the One Health movement include One Health Commission and the One Health Initiative. The CDC also includes “One Health” as an important public health approach. Several large projects also originate from the AVMA-accredited colleges of veterinary medicine.
Existing One Health projects
Removing exclusion fencing
Veterinary cordon fences exclude wild herbivores from rangelands throughout southern Africa to reduce the transmission of foot-and-mouth disease between wild herbivores and livestock (Gadd et al. 2012). Foot-and-mouth disease is nonlethal to livestock, but has painful symptoms. Wild animals typically carry the disease asymptomatically (Young et al. 1972, Vosloo et al. 2007).
Exclusion fencing may degrade wild animals’ quality of life more than foot-and-mouth disease itself. Exclusion fencing fragments home ranges and disrupts migration routes. When their movement is constrained, animals cannot escape from potential harms as easily. As a result, cordoned animals more frequently die from starvation, dehydration, electrocution, and entanglement (Gadd et al. 2012, Lindsey et al. 2012).
The costs of exclusion fencing also permeate the socio-economic climate in Africa (ibid, ibid). Outbreaks of foot-and-mouth disease still occur due to fencing failures or mistakes in the food regulatory chain (Thomson et al. 2018). Meat from exposed livestock cannot be sold (ibid), a burden that is largest for low-income producers (Whitmee et al. 2015).
Exclusion fencing does not eradicate poverty from foot-and-mouth disease, and it creates a number of welfare problems for vast numbers of wild animals. The One Health solutions are two-fold: change the regulations for beef so that the process is more humane for people and livestock (MacKenzie et al. 2013), and dismantle fencing. Deaths by entanglement, electrocution, and exclusion-related starvation and dehydration are reduced, and restoring migratory routes improves animals' abilities to act on their behavioral preferences (Gadd et al. 2012, Mellor and Beausoleil 2015, Allen et al. 2018).
Eradicating tuberculosis
Tuberculosis, a severe bacterial infection of the lungs, circulates among human, livestock, and wildlife hosts. Many low-income countries have not controlled tuberculosis. In Tanzania, for example, as many as 16% of all people have had the disease (Travis et al. 2019), as have 1%-13% of cattle, depending on the region (Katale et al. 2013).
An active One Health project has identified buffalo (Syncerus caffer) as a major disease reservoir (Travis et al. 2019, Roug et al. 2020a) and revealed that habitat loss and intensive cattle grazing in and around natural areas have exacerbated transmission by bringing wild buffalo in more frequent contact with people and domestic animals (Kirksey 2012, Roug et al. 2020b).
Rangeland degradation appears to be an overarching problem contributing to poor health from tuberculosis. Following the One Health approach, reducing habitat loss may be a single-source solution that benefits the quality of life of many people and animals.
Surveilling emerging infectious diseases
“Emerging infectious diseases” is an umbrella term for pathogens that are newly discovered, recently evolved, or occupying new ranges or hosts (Rachowicz et al. 2005, Dazak et al. 2000, Bird and Mazet 2018). Emerging infectious diseases tolerated in one host can become epidemics in others — resulting in extinctions, suffering, or economic damage (Rachowicz et al. 2005, Barrett 2014, Zukai 2014). One Health projects to address this include the establishment of a global disease surveillance system with the capacity to respond to these health threats in real time (Bird and Mazet 2018).
This surveillance network is applied to diseases that primarily affect wild animals. Wild rhinoceroses and giraffes have recently displayed novel ulcerative skin infections. The cause in rhinos is thought to be a roundworm, but for giraffes it remains a mystery (Mutinda et al. 2012, Muneza et al. 2016). Disease monitoring efforts like these are a promising tool for learning more about diseases that primarily affect wild animals.
Uncounted numbers of diseases circulate among different wildlife species, but because of the lack of information on diseases that do not (yet) affect people, we have almost no information on the costs these unmonitored pathogens have for wild animals (Dazak et al. 2000). Understanding disease dynamics helps people stop the spread of wildlife diseases to new populations and intervene to restore good health to wild animals currently burdened by disease.
Future research to improve wild animal welfare
Target the worst diseases
One Health has been used many times to address diseases in wild animals. But in some of these instances, wild animals are merely asymptomatic carriers of these diseases. Pathogens that cause painful and prolonged symptoms in wild animals more significantly decrease their welfare (Beausoleil et al. 2012, Allen et al. 2018).
These diseases should be prioritized. Research that compares the relative severity of emerging infectious diseases — regardless of whether the diseases are transmissible to livestock or people — could be a promising way to apply One Health’s disease surveillance network to address wild animal welfare.
It is also important to increase our capacity to address wildlife health issues so that we can treat the prolonged and painful diseases once we discover them. Wildlife health facilities are also a valuable repository of information about wildlife health issues on the ground (Loyd et al. 2017). Expanding these facilities helps us understand which diseases are most common or cause the most suffering.
Target common animals
Common species such as urban animals, livestock competitors, and agricultural pests tend to be of minimal conservation concern because of their abundance. But welfare efforts targeting common animals are likely to be most cost effective per capita because interventions can be distributed to vast numbers of individuals.
Pigeons heavily populate human-animal interfaces — areas in the landscape where human and non-human animals cohabitate (Kirksey 2012, Pacini-Ketchabaw and Nxumalo 2015). Because pigeons live in cities, they are vulnerable to avoidable and devastating anthropogenic health problems (Murray et al. 2019).
Targeting common animals like pigeons has strong potential to ameliorate welfare issues due to poor health on a large scale. Citizen science data from New York City pigeons indicate that on average, 165 pigeons annually are poisoned by lead (Cai and Calisi 2016). There is no reason to expect this rate to dissipate with time. It is also a minimum estimate because the data is sourced from one wildlife rehabilitation center. If this rate is reflected in all 33 megacities, then at least 5445 pigeons are poisoned each year globally.
Conclusion
Human, animal, and environmental health are linked. It is essential to address healthcare in wild animals to ensure they have good welfare. Veterinarians and wildlife health scientists who follow the One Health approach care about wildlife health for the sake of wild animals’ welfare, not just because wildlife health issues spill over to people.
One Health continues to make strides toward building a global healthcare system for people, domestic animals, and wild animals. Though One Health projects tend to focus on implications for livestock and human health, there is potential for expansion into a range of wild animal welfare issues. A maximally beneficial welfare intervention would target common animals and their most prolonged and painful health issues.
One Health represents an unusual example of institutions and funding dedicated to improving wildlife health and engages a wide range of scientists and veterinarians. A wild animal welfare project that demonstrates co-benefits to livestock and people could similarly engage these practitioners by demonstrating that improving the welfare of wild animals is an important, multi-dimensional societal issue.
Why cause of death matters for wild animal welfare
In part one of this series, Luke Hecht introduces approaches to studying wild animals’ causes of death, with the goal of making work in this field maximally useful for understanding wild animal welfare.
The most fundamental reason for viewing death as a bad thing is that it deprives individuals of future experiences, assuming those experiences would have been predominantly positive. However, the process of dying is also often painful in itself, and an individual’s death can have additional negative impacts—emotional and material—on others, including family and the broader population.
The costs of a death depend on its cause. Humans are willing to sacrifice periods of healthy life to avoid especially unpleasant deaths, and see various causes of death as more fearsome than others (Sunstein 1997; Chapple et al. 2006). Of course, people’s perception of how much suffering particular deaths would entail may be biased by things like squeamishness; they may assume that because a death is gruesome it must be especially painful. The terminal stages in the process of dying, during which suffering presumably outweighs pleasure, comprise a small fraction of most humans’ lives. Willingness to trade years of healthy life to obtain a quicker, less painful death implies that either the original manner of death must be extremely bad, or that we have an exaggerated sense of how bad dying is.
As recently as the 19th century, only around half of newborns could expect to survive to adulthood (Riley 2005). Many human cultures have had traditions of only conferring names on children when they reached a certain age, potentially because it was understood that most would not survive that long (but that if they did, they would have a decent prospect of reaching adulthood) (Lancy 2014). For example, birth registration was not a legal requirement in the United Kingdom until 1874 (ONS 2015). As average lifespan increases, the dying process represents an ever smaller proportion of human life.
While human childhood mortality has declined dramatically throughout much of the world, most wild animals still die at a young age relative to the longest-lived members of their own species (Figure 1). For these animals, the process of dying may actually represent a substantial portion of their lifetimes, suggesting that, in addition to knowing the most likely causes of death for entire populations, it would be especially valuable to know which manners of death are common among juveniles in specific wild animal populations.
Figure 1: A boxplot showing life expectancy as a percentage of a species’ maximum lifespan for 152 populations of fish (n=16), birds (n=54), mammals (n=72) and reptiles (n=10). Life expectancies were calculated from models found in the COMADRE database (Salguero-Gomez et al. 2016), and maximum lifespans were obtained from AnAge (De Magalhães et al. 2005). Across major vertebrate classes, most individuals live to only 10-30% of the age of the oldest known individuals of their species.
“Cause” of death can be interpreted in at least two different ways: “manner” of death, or “ultimate cause” of death. Both matter for wild animal welfare, but they can be difficult to disentangle in practice. For example, an animal who died during a forest fire may have died by burning or asphyxiation. Other animals may die in the immediate aftermath of a forest fire from dehydration or infected burn wounds. The welfare impact of these different manners of death would likely vary, and it is possible in theory to rank their welfare impacts based on the intensity and duration of suffering leading up to death (Figure 2; Sharp and Saunders 2011). Learning about the precise manner in which wild animals die can help us prioritize hazards to protect them from to reduce instances of the most extreme suffering. On the other hand, it is often easier to estimate the number of animals who died due to an ultimate cause - such as forest fires - than to break this down into specific manners of death. Learning about an ultimate cause of death, and the number of deaths resulting from it, is also useful for prioritizing welfare interventions directed at extending good lives.
Figure 2: An example scheme for grading the welfare impact of different manners of death, taking into account both the severity of suffering inflicted and the length of time it takes for the animal to die. Adapted from work by Sharp and Saunders (2011) on wild animal culling methods.
To minimize the suffering animals experience in the wild, we need to understand how and why wild animals die, paying particular attention to the most numerous experiences: that is, the deaths of juvenile animals belonging to common species. The rates of different causes of death are also valuable to know because even if dying turns out to be a minor contributor to lifetime welfare, or the difference in severity between manners of death is relatively low, substituting one manner of death for another less painful one could improve individual welfare without affecting population size, minimizing the number of variables we need to consider to determine whether an intervention is worth implementing.
In a series of posts to follow, I will review the methods available for studying causes of wild animal deaths and present a selection of published data on cause-specific mortality rates. Finally, I’ll describe some original modeling by former Wild Animal Initiative Intern Anthony DiGiovanni that explicitly considers how cause of death may change along with the size and demography of wild animal populations.
References
Sunstein, C. (1997). Bad deaths. Journal of Risk and Uncertainty, 14(3), 259-282.
Chapple, A., Ziebland, S., McPherson, A., & Herxheimer, A. (2006). What people close to death say about euthanasia and assisted suicide: a qualitative study. Journal of Medical Ethics, 32(12), 706-710.
Riley, J. C. (2005). Estimates of regional and global life expectancy, 1800–2001. Population and Development Review, 31(3), 537-543.
Lancy, D. (2014). “Babies aren’t persons”: A survey of delayed personhood. In H. Otto & H. Keller (Eds.), Different Faces of Attachment: Cultural Variations on a Universal Human Need (pp. 66-110). Cambridge: Cambridge University Press.
Office for National Statistics. (2015). How has life expectancy changed over time? Retrieved from https://www.ons.gov.uk/peoplepopulationandcommunity/birthsdeathsandmarriages/lifeexpectancies/articles/howhaslifeexpectancychangedovertime/2015-09-09.
Sharp, T., & Saunders, G. (2011). A model for assessing the relative humaneness of pest animal control methods. Canberra, Australia: Department of Agriculture, Fisheries and Forestry.
De Magalhaes, J. P., & Costa, J. (2009). A database of vertebrate longevity records and their relation to other life‐history traits. Journal of Evolutionary Biology, 22(8), 1770-1774.
Salguero‐Gómez, R., Jones, O. R., Archer, C. R., Bein, C., de Buhr, H., Farack, C., ... & Römer, G. (2016). COMADRE: a global database of animal demography. Journal of Animal Ecology, 85(2), 371-384.
Assessing biomarkers of aging as measures of cumulative animal welfare
Will Bradshaw
Will Bradshaw reviews the existing empirical support for the use of biomarkers of aging as a measure of cumulative welfare, discusses the prerequisites of applying the method, and explores a number of important caveats that may limit its applicability.
Summary
In order to determine which conditions provide the best overall quality of life for nonhuman animals, it is important to be able to measure their cumulative welfare experience. The ideal measure of cumulative welfare would be comprehensive, objectively measurable, and easy to transfer across species; however, existing approaches fall far short of this ideal. Recent academic work has suggested that measures of biological aging could provide a highly promising alternative measure of cumulative welfare, which comes much closer to meeting these ideal goals.
Here, I review the existing empirical support for the use of biomarkers of aging as a measure of cumulative welfare, discuss the prerequisites of applying the method, and explore a number of important caveats that may limit its applicability. Many of these caveats are particularly applicable to the study of wild animal welfare, though some may also be important in domesticated contexts.
Overall, despite some important potential weaknesses, biomarkers of aging are likely to represent an important step forward in the assessment of cumulative animal welfare, which could potentially help resolve some important long-running uncertainties and disputes in the animal welfare movement. Wild Animal Initiative recommends that both researchers and funders take note of these new techniques, and consider how best they can develop them further or apply them in their own domains of expertise.
We need a good way of measuring cumulative animal welfare
While single experiences can be acutely positive or negative, what matters more from a welfare perspective is the lasting cumulative impact of these experiences.
— Bateson & Pourier 2019 1
Over the course of their life, an animal will undergo various positive and negative experiences. Some of these experiences will primarily affect welfare in an acute, short-lived manner, while others will have significant long-lasting welfare effects. The overall welfare state of an animal will be determined by the cumulative effect of all the experiences they have faced in the course of their life to date.
In order to improve animal welfare, we need to know what affects it. In order to improve animal welfare effectively, we need to know which factors have the greatest total effect on animals’ cumulative wellbeing. Actually measuring cumulative wellbeing, however, is highly challenging, and our existing methods for doing so frequently rely on crude proxies or error-prone anthropomorphic judgements. To make matters worse, the welfare effect of a given experience is frequently complex, species-specific, and non-obvious to humans:
- Species of fish which are naturally solitary exhibit stress indicators upon overcrowding, while schooling fish become stressed if raised at unnaturally low densities 2.
- Exposure to environmental ultrasound frequencies that are undetectable to humans causes depression-like symptoms in laboratory rats and mice 3.
- The flickering of some fluorescent lights at frequencies above the human flicker-fusion rate raises stress-hormone levels in starlings 4.
- The stress-hormone levels induced in captive-bred lizards by different experimental procedures can differ dramatically from how stressful human observers think the procedures are, with one controversial procedure found to generate much lower levels of stress hormone than the “less-stressful” procedure that replaced it 5.
Which specific stimuli are important for an animal’s welfare is therefore very difficult to predict a priori, and the use of anthropomorphism to make these predictions is fraught with danger.
Even if it is possible to determine that a given experience is acutely positive or negative for an animal, it is not obvious how to convert these acute measurements into measurements of lasting impacts on welfare. Experiences with similar acute effects may have dramatically differences on long-run wellbeing: one stressor may involve temporary pain or result in a temporary spike in stress-hormone levels but have no long-term effects, while another might significantly contribute to long-term stress levels. In some cases, the short- and long-term welfare impacts of an exposure may even be of opposite sign: some acute stresses can be beneficial in the long-term, while the short-term pleasure of sugary food might be outweighed by the long-term pain caused by tooth decay and weight gain 1. Worse, the relationship between short-term and long-term welfare impacts is likely to vary significantly based on species, subspecies, chronological age, past experiences, and individual genetic variation, making the long-term welfare effect of an acute experience even more difficult to predict.
A good measure of cumulative affective experience 6 is therefore vital to the study of animal welfare. However, developing a cumulative-welfare metric which is sensitive, easy to measure, captures all or almost all of the relevant effects on welfare, and can be transferred with relative ease across species has proven to be highly challenging 1. Existing measures include chronic physiological markers such as resting stress-hormone levels or bodyweight, acute behavioral measures such as the presence of stereotypies, and high-level behavioral measures such as depressive symptoms or cognitive biases 1; other measures combine multiple different sources of evidence, often alongside acute welfare indicators and/or subjective welfare assessments by a trained practitioner. However, all of these methods have serious drawbacks: physiological measures often lack sensitivity and specificity (i.e. they do not always reliably correlate with affective state), stereotypies are highly species-specific and often difficult to interpret, and the behavioral tests required to measure cognitive biases and mood must be developed and validated independently for each species and often require extensive animal training. Combined measures, meanwhile, rely on difficult-to-test assumptions about how different metrics should be combined and weighted to assess overall cumulative wellbeing.
In the ideal case, the many and varied inputs into cumulative animal welfare would be captured by a single, objectively measurable metric, which could then be used as a single readout of cumulative wellbeing for many different species of animals. In the rest of this article, I will review and discuss the evidence supporting a new, surprisingly good candidate for this “objectively measurable common currency” 1 of wellbeing: biomarkers of the aging process.
Biological aging and cumulative animal welfare
As we get older, our bodies decay. In various ways, our cells and tissues progressively accumulate increasing levels of damage and dysregulation, leading at the whole-organism level to a decline in functionality, an increase in mortality, and a decrease in reproductive output 7. These deteriorative processes, which occur in broadly similar ways in many different animal species 8, are collectively known as aging.
Among researchers studying the biology of aging, it is well-known that simple chronological age is a less-than-perfect measure of the aspects of aging we tend to care about. In humans, individuals of the same chronological age often differ substantially, both in how old they appear to others (degree of graying, wrinkles, stooping, etc.) and in their age-related health outcomes 9. It is therefore useful to separate the concepts of “chronological age” (time since conception or birth) and “biological age” (degree of age-related change/deterioration in appearance, health, or functionality) 9, 10. So-called “biological aging clocks”, which incorporate a variety of different types of biological data, are an active area of research in the study of human aging, and the best such clocks can predict health and other outcomes much more accurately than chronological age alone 10.
The biological age of an individual depends on their chronological age, genetic background, and environmental history: depending on their genes and experiences, two individuals of the same chronological age can differ substantially in their biological age. A striking example of this is smoking, which produces a variety of aging-like symptoms and has recently been shown to substantially increase biological age in young humans 11. However, biological age is affected by a wide variety of chemical, psychological and social stimuli, many of which are also known to have important effects on an individual’s wellbeing. To take just one example, a wide variety of negative experiences (including anxiety, depression, childhood trauma, chronic pain, and various forms of stress) are associated with reduced telomere length in humans, while positive lifestyle factors are associated with longer telomeres 12, 1, 13, 14. Despite important differences in telomere biology between species, a variety of stress manipulations (including social isolation, sleep disruption, injection of stress hormones, and crowding) have also been found to accelerate telomere attrition in various nonhuman animals, including wild and laboratory mice, chickens, starlings, and various other bird species 12.
In addition to this and other empirical data, there are good theoretical reasons to expect the rate of biological aging to correlate with the cumulative affective experience of an individual 1. Evolutionarily speaking, the affective state of an experience serves to motivate an animal to seek or avoid similar experiences; hence, affectively negative experiences tend to be those that reduce animal’s fitness, while affectively positive experiences tend to be those that increase it. An important way in which an experience can decrease fitness is by causing or contributing to some sort of damage or dysregulation in the body; hence, experiences which contribute to damage or dysregulation will typically be perceived as aversive to the organism, while those that prevent or reverse damage (or have no effect on damage but are positive for some other reason) will be perceived as attractive. In general, therefore, there is good reason to expect physiologically-damaging experiences to be affectively negative, and vice versa 15, with a similar connection between physiologically-protective experiences and positive affect. Since aging is characterized by the progressive accumulation of various forms of physiological damage, this suggests that there is good reason to expect the affective valence (i.e. the positivity or negativity) of an experience to also generally correlate with its effect on biological age.
There are, therefore, both empirical data and theoretical arguments suggesting a relationship between cumulative affective experience and aging. If this relationship exists, the biological age of an individual relative to their chronological age could be used to assess that individual’s cumulative welfare experience up to that point. If further testing bears this out, there are several reasons to expect biological age to be a particularly valuable tool for assessing wellbeing:
- It is highly general, including all (or almost all) causes of stress and wellbeing experienced by the animal, including those not obvious or perceptible to humans
- It is cumulative, giving a readout of the total affective history of an individual
- It is objectively measurable, with well-established biomarkers already known for a number of species
- Finally, it is plausibly relatively phylogenetically neutral: as aging is a general phenomenon shared by very many species, whose measurement does not generally rely on anthropomorphic judgements, it can potentially be used to investigate welfare in many different animal groups.
Given these potential advantages, how might we go about actually measuring biological age?
Measuring biological age in non-human animals
In any given species, the aging process will manifest itself in a plethora of different phenotypes, many of which can be used to try to quantify biological aging. In humans, a wide variety of biological readouts have been used as biomarkers of aging, including telomere length and attrition rate, DNA methylation patterns, gene expression profiles, changes in neuroanatomy, proteomic and metabolomic changes, and various composites of clinically relevant symptoms 1, 16, 17. While many of these biomarkers predict health outcomes better than chronological age, they often reflect different aspects of the aging process and do not always correlate well with one another 1, 16. Combining different measures (e.g. with machine-learning-based prediction tools) can overcome these problems and improve the ability of a biological aging measure to predict health outcomes 17, 11.
As different biomarkers of aging track different aspects of the aging process, they may differ in how well they measure cumulative subjective wellbeing. A good biomarker of cumulative experience should provide a single, continuous, easy to measure readout that responds in opposite directions to positive and negative affective experiences in a cumulative and dose-dependent manner 12, 1. Different biomarkers will also differ in the money, expertise and time required to obtain good measurements, and in the ease with which they can be validated in a new context. As nonhuman animals, unlike humans, cannot explicitly self-report their subjective experience, validation of a new potential biomarker’s relationship with wellbeing is much more challenging in these species; as a result, the ease and reliability with which a biomarker can be transferred between species is an additional important consideration when choosing how to measure biological age in an animal welfare context.
Overall, I would expect more complex and multi-modal measures to provide a more accurate, precise and thorough measure of biological age and so give a better idea of an animal’s cumulative experience. On the other hand, I would expect these complex measures to be more expensive and time-consuming to obtain for each individual, and less transferable between species.
For some widely-used farmed species and experimental model organisms, it may well be worth developing sophisticated species-specific methods of measuring biological age and hence cumulative welfare; however, in contexts where resources are highly limited and/or the number of species of concern is large, cost and transferability concerns are likely to mitigate in favour of simpler, faster, cruder measures 18. Bateson & Pourier 12, 1 suggest telomere length and hippocampal volume as two metrics that are simple, well-defined, and likely to retain validity across a wide variety of vertebrate species; further work may reveal other promising candidates. However, when using such very simple readouts of biological age, it is important to remember that they may give a significantly more partial and inaccurate reading than more sophisticated measures, and to seek to develop such improved measures where feasible.
A concrete example: the welfare effect of crowding on farmed fishes
Many farmed fish are kept at very high densities, in a manner which often appears to be detrimental to their welfare 2. The potential welfare effects of crowding are many and varied, including social stresses, reduced water quality, and increased disease transmission. However, the actual welfare effect of a given level of crowding will vary between fish populations, depending on the level of crowding and water quality they are adapted to cope with, the robustness of their immune systems, and whether they have been vaccinated, among other factors. It would be useful to measure the total cumulative welfare effect of different crowding regimes on different species, and to know the degree to which other interventions such as vaccination mitigate any crowding-induced welfare reduction. Biological age provides an ideal means of addressing these questions.
To apply this method, we would need some sort of biological aging clock for each of the fish species of interest, as well as some way of keeping track of the chronological age of each individual. Once these two methods are in place for each species, the experiment is simple: simply raise populations of fish at different levels of crowding, sample the biological ages of individuals in each population, then compare the biological ages of chronological-age-matched individuals from different conditions. Those populations exhibiting the highest biological age relative to their chronological age would be taken as experiencing the lowest levels of cumulative welfare.
Plots showing speculative results of a hypothetical experiment investigating the effect of crowding on fish welfare, indicating that biological age accumulates slowest (indicating highest welfare) at low densities for solitary fish and middling densities for schooling fish, with a positive welfare effect of vaccination. The shape of the curves is arbitrary.
To make up some totally speculative results, these biological aging data might indicate that naturally solitary fish exhibit significant welfare declines from any level of crowding, while schooling fish have a preferred crowding level and experience reduced welfare above or below this level. These welfare declines may or may not be abrogated by interventions such as vaccination, better water filtration, or changes in feeding schedule. By collapsing all (or most) of an animal’s welfare experience into a single, objective, cumulative measure, many different experiments of this kind could be performed quickly and efficiently, providing a more comprehensive picture of the welfare effects of crowding on fish wellbeing.
While the idea that crowding is bad for fish welfare may not be particularly controversial, this approach would allow researchers to empirically quantify how bad that effect is compared to other aspects of a farmed fish’s life, and assess the efficacy of different interventions (such as vaccination or water oxygenation) for mitigating that welfare impact. The same technique could be applied to help resolve active empirical controversies in the animal welfare movement, such as the relative welfare levels of caged vs cage-free chickens, the relative importance of water oxygenation in the welfare of farmed fishes, or the net welfare impact of predator reintroduction on prey species.
Limitations and caveats
While I was initially skeptical about the applicability of biological aging markers as measures of animal welfare, I have generally been convinced that this represents a novel and important advance in the field. Nevertheless, there are a number of important limitations or difficulties I anticipate in actually applying the method, which I think it is important to be aware of and mitigate where possible. I have divided these limitations into two categories: contexts where the prerequisites of applying the method may be difficult or expensive to obtain, and contexts where the link between aging and welfare may be weakened or broken entirely.
Difficulties in application
The need for biological aging clocks
In order to use biological aging as a cumulative welfare measure in a given species, we need some kind of biological aging measure for that species. As discussed above, these range in complexity from simple metrics like telomere length to highly complex machine-learning-based predictors, and there is likely to be a tradeoff between the accuracy and comprehensiveness of a measure on the one hand and its affordability and transferability on the other. As funding is very limited in many animal welfare contexts, it is likely that simpler, cheaper metrics that can be transferred between species with relatively little validation will be preferred; however, it is important to remember that these may only provide a partial measure of biological age.
The best biomarkers of aging to use for these experiments will depend on the species being tested and its relationship to other well-validated model systems. In many vertebrate species, and probably most mammals, the markers established in humans and laboratory mice are likely to be the best option. Conversely, in species very distantly related to humans the validity of these markers may be limited: insects, for example, are largely post-mitotic in their adult form (limiting the usefulness of telomere attrition as a biomarker) and have very different neuroanatomy from vertebrates (preventing the use of hippocampal volume). If it is desirable to apply these aging-based methods to assess welfare in these species, alternative biomarkers (such as accumulation of fluorescent advanced glycation end products in Drosophila 19) will need to be developed and validated as welfare measures, substantially increasing the upfront cost.
Experimental controls
While biological aging is a promising measure of cumulative welfare, it is important to remember that welfare is not the only thing affecting biological age. Most obviously, chronological age has a very strong effect on biological age, and studies should always compare age-matched individuals when possible. Genetic variability is also an important factor: many species exhibit substantially different lifespans in different populations, and polymorphisms within a population can also have a substantial effect. As a result, aging-based welfare measures will be most reliable in contexts where all individuals are genetically homogeneous, or at least where there is no systematic difference in genetic composition between different experimental groups. Finally, there should of course be as little systematic difference in environment as possible between the groups being compared, other than whatever exposure is being investigated for its welfare effects.
Of these control requirements, the need for genetic comparability between experimental groups is the most frustrating, as it appears to exclude a lot of factors that are widely thought to be important for animal welfare, particularly in domesticated contexts where animals’ genotypes have been substantially modified by humans through selective breeding. On the face of it, biological-aging methods seem to be unable to address this, as the groups being compared are not genetically comparable. However, given the importance of genetic effects on welfare in many contexts, any way to overcome this limitation would be very useful, and further investigation on this front seems quite valuable in expectation.
Limitations to validity
Death and other acute events
In many cases, a large portion of the suffering experienced by an animal, whether domesticated or wild, is suspected to take place acutely at the moment of death. If this death is sufficiently gruesome, the suffering so engendered could potentially outweigh the entire net welfare of a life that is otherwise worth living. Despite its importance, however, it seems unlikely that the suffering entailed by dying would be adequately reflected in aging-based measures of cumulative welfare. For one thing, there would be no opportunity for the negative experiences associated with the animal’s death to be reflected as a subsequent increase in the rate of biological aging; for another, the extensive physiological damage resulting from death would prevent an accurate postmortem assessment of biological age in many cases. Hence, an important limitation of aging-based methods of measuring welfare may be their inability to incorporate the affective experience of dying.
A similar concern might apply, albeit to a much lesser extent, to other highly acute exposures, i.e. those with large but short-lived effects on welfare. Many of these will be reflected in ongoing cumulative welfare to some extent (e.g. as physical or psychological trauma in the case of negative events), but it’s not clear to me that the cumulative readout of welfare given by aging biomarkers will always incorporate them adequately. More research may be needed here.
Differences between juveniles and adults
In both wild and domesticated contexts, many of the animals of greatest concern from a welfare perspective are juveniles who have not yet reached reproductive maturation. These immature individuals are often much more numerous than adults, and have less chance to accumulate positive experiences to outweigh the pain of dying.
For animals that die shortly after birth or hatching, the cumulative welfare of their lives is likely to be dominated by the affective experience of dying, and this experience will not be adequately reflected in biological aging markers. aging-based approaches therefore seem unable to effectively address the welfare of these individuals; on the other hand, it seems likely that any measure of cumulative welfare will run into the same problem.
The situation for individuals that have relatively long lives as juveniles is more complex. In many species, juvenile and adult individuals differ substantially in their biology, and the question of whether juveniles are “aging” is somewhat fraught. There are certainly dramatic changes taking place over the course of development, some of which could be interpreted as an accumulation of damage: telomeres, for example, shorten rapidly during the period of juvenile growth 20. On the other hand, many clinical biomarkers of aging do not begin to accumulate until adulthood 21. This means that the applicability of biological-aging measures to juveniles depends on the specific biomarkers being used, and different markers are likely to be most appropriate for measuring juvenile vs adult wellbeing 22.
Animals very different from humans
The evidence supporting the use of biological aging markers as measures of cumulative welfare falls into three broad categories: a theoretical, evolutionary argument linking the affective status of an exposure to its effect on aging via its effect on somatic damage; extensive empirical research in humans associating biological aging markers with directly reported affective mood (e.g. stress, depression or anxiety) and experiences known to impact mood (e.g. trauma, pain, exercise, and sleep); and more limited empirical research in animals linking these markers to experiences that are both plausibly affectively relevant and associated with other widely-used welfare measures.
The second of these, empirical data on humans, is particularly important, as only humans are capable of directly reporting their affective state to human researchers and so directly confirming a link between biological aging and welfare. As one moves away from humans in terms of the species under investigation, the less weight can be put on this source of evidence in support of this link, and the more one has to rely on the first and third sources of evidence outlined above. The more distant and dissimilar a species is from those species in which aging-base techniques have been empirically studied, the more our confidence in those techniques should decrease towards the level of confidence we have in the theoretical argument alone. This poses an issue, since the vast majority of animals on the planet fall into this category.
There are two particular groups of animals for which I think the existing empirical data provides relatively little support for aging-based welfare measures: invertebrates, and those animals (both vertebrate and invertebrate) whose pattern of lifetime aging differs substantially from that of humans. The reasons for skepticism in the first case are clear: invertebrates are very different from vertebrates in many aspects of their biology, differ substantially from mammals in terms of their biomarkers of aging (see above), are very diverse amongst themselves, and are almost totally unstudied as objects of welfare concern. My concerns about the second group, however, are likely to be less clear to someone outside the aging field, and I will try to briefly explain my reasons below.
Typically, under simple assumptions that are frequently roughly met in real animals, we expect to see mortality progressively increase and fecundity progressively decrease with time after reproductive maturation 23. Many animals, including humans and nearly all common domesticated species, follow this pattern, but this does not apply universally to all animals. Some species, most famously the green hydra 24 and more recently the naked mole rat 25, do not appear to age at all, while some (including various corals, reptiles and amphibians 8) seem to “age in reverse”, exhibiting declining mortality with age until death.
These differences in life history could pose major problems for the use of biological aging markers as a measure of cumulative welfare in these species. To begin with, it isn’t clear how to define the concept of biological age, let alone measure it, in an animal that does not age in any conventional way. Some subset of conventional biomarkers of aging may still accumulate with time in these species, but that subset is likely to differ from taxon to taxon depending on what biological methods they have used to overcome the aging process. Worse, even the theoretical argument in support of aging-based welfare measures in these species may be greatly weakened: for an animal to exhibit no aging or even reverse aging over a prolonged time period, they must be either extremely resistant to somatic damage or have extremely good mechanisms in place to repair that damage, meaning the relationship between damaging (and therefore aversive) experiences and aging may be largely or entirely severed.
These issues will pose little difficulty to researchers and activists concerned with the welfare of agricultural, experimental or other captive animals, most or all of which, to my knowledge, follow the conventional pattern of aging exhibited by humans, mice, and Drosophila. However, they could turn out to be significant for researchers interested in quantifying and improving the welfare of wild animals, who will inevitably have to tackle the welfare of large numbers of animals very different from humans. The extent to which these “unconventional” life histories are widespread in the natural world is unclear to me at present, and could be an important factor affecting the applicability of these methods in certain contexts.
Conclusion
Finding better methods with which to quantitatively measure the cumulative welfare experience of nonhuman animals would represent a major advance in the study of animal welfare. As a potential route to a better and more objective measure of cumulative welfare, biomarkers of aging are potentially very promising.
Many of the caveats I outlined above are educated guesses and may well turn out to be circumventable with sufficient thought and care. If they are not, how serious a problem would this pose for the practical usefulness of this method? In the case of domesticated animals, an inability to compare groups differing systematically in their genetics or adequately incorporate the badness of death are all significant limitations, but would still leave us with a tool which could be gainfully applied in many important contexts. For wild animals, the issues are more serious: there are vastly more species for which we would have to develop methods of measuring biological age, it is much harder to perform well-controlled longitudinal experiments, and many more of the animals of concern fall into categories for which I am more skeptical about the theoretical applicability of the method. A further concern is that measuring chronological age accurately is often difficult for wild animals, potentially undermining one of the foundations of aging-based welfare measures. Nevertheless, of all the methods we might think of for measuring the cumulative welfare of wild animals, biomarkers of aging seem to be among the least hopeless, and among those most worth developing further in the hopes of overcoming some of these pervasive issues.
Overall, I am currently very optimistic about the value of applying these methods in domesticated contexts, and cautiously optimistic about applying them to wild ecosystems. I would recommend that researchers interested in the welfare of either domesticated or wild animals take note of these techniques and consider their applicability in their own domains, and that funders in this space seriously consider funding their further development and application to new contexts. On a meta level, I think the surprisingly strong applicability of techniques from the biology of aging to animal welfare science should encourage us all to look for innovative, unexpected and interdisciplinary ways to help nonhuman animals. As is often the case in science, the crucial insights and discoveries may not be at all where we expect them to be.
Acknowledgements
Professor Melissa Bateson, the author of the key sources for the report, generously read the draft of this report and provided crucial feedback on several important points.
References
Bateson, M., & Poirier, C. (2019). Can biomarkers of biological age be used to assess cumulative lifetime experience? Anim. Welf. 28: 41-56. doi: 10.7120/09627286.28.1.041 ↩︎ ↩︎ ↩︎ ↩︎ ↩︎ ↩︎ ↩︎ ↩︎ ↩︎ ↩︎ ↩︎
Ashley, P. J. (2007). Fish welfare: current issues in aquaculture. Applied Animal Behaviour Science 104 (3-4): 199-235. ↩︎ ↩︎
Morozova, A., et al. (2016) Ultrasound of alternating frequencies and variable emotional impact evokes depressive syndrome in mice and rats. Progress in Neuropsychopharmacology and Biological Psychiatry 68: 52-63. ↩︎
Smith, E.L., et al. (2005) Effect of repetitive visual stimuli on behaviour and plasma corticosterone of European starlings. Animal Biology 55: 245-258. ↩︎
Langkilde, T., & Shine, R. (2006). How much stress do researchers inflict on their study animals? A case study using a scincid lizard, Eulamprus heatwolei. Journal of Experimental Biology 209 (6): 1035-1043. ↩︎
Throughout this piece I am assuming that welfare is synonymous with affect, i.e. with subjectively positive and negative experiences. This is not an uncontroversial position, and there are many in the animal welfare field who prefer a concept of welfare which incorporates both affective wellbeing and physical health, or even avoids questions of subjective experience altogether 26. While I’m skeptical about this, it’s worth noting that measures of cumulative wellbeing are also essential when using such a definition, so it doesn’t necessarily change many of the conclusions of this report. ↩︎
López-Otín, C., Blasco, M. A., Partridge, L., Serrano, M., & Kroemer, G. (2013). The Hallmarks of Aging. Cell 153 (6): 1194-1217. ↩︎
Jones, O. R., et al. (2014). Diversity of ageing across the tree of life. Nature 505 (7482): 169. ↩︎ ↩︎
Jia, L., Zhang, W., & Chen, X. (2017). Common methods of biological age estimation. Clinical Interventions in Aging 12: 759-772. ↩︎ ↩︎
Horvath, S., & Raj, K. (2018). DNA methylation-based biomarkers and the epigenetic clock theory of ageing. Nature Reviews Genetics 19 (6): 371-384. ↩︎ ↩︎
Mamoshina, P., et al. (2019). Blood Biochemistry Analysis to Detect Smoking Status and Quantify Accelerated Aging in Smokers. Scientific Reports 9: 142. ↩︎ ↩︎
Bateson, M. (2016). Cumulative stress in research animals: Telomere attrition as a biomarker in a welfare context?. BioEssays 38 (2): 201-212. ↩︎ ↩︎ ↩︎ ↩︎
Pepper, G.V., et al. (2018) Telomeres as integrative markers of exposure to stress and aversity: a systematic review and meta-analysis. Royal Society Open Science 5: 180744. ↩︎
It’s important to stress here that the great majority of research into the association between lifestyle and telomere length is cross-sectional and correlational: individuals with greater exposure to adversity have shorter telomeres. This finding is robust, but not causal: it doesn’t demonstrate that these adverse experiences cause shorter telomeres. Evidence from longitudinal studies, for example on smoking 27, tend to find a much smaller effect; more on this later. ↩︎
One major category of negative experiences which do not appear to be directly damaging (but are nevertheless important to an animal’s wellbeing) are social experiences. For many reasons, an animal’s social status and relationships are very important to their survival and reproduction, but are not typically the direct cause of bodily damage. However, negative social experiences (low status, rejection by mates, ostracism) do give rise to significant levels of stress in many species, and this stress is well known to be physiologically damaging. ↩︎
Belsky, D. W., et al. (2017). Eleven telomere, epigenetic clock, and biomarker-composite quantifications of biological aging: do they measure the same thing? American Journal of Epidemiology 187 (6): 1220-1230. ↩︎ ↩︎
Cole, J. H. et al. (2018). Brain age predicts mortality. Molecular Psychiatry 23 (5): 1385-1392. ↩︎ ↩︎
Melissa Bateson (pers. comm.) points out that a “complex and multi-modal” measure of biological aging does not necessarily entail expensive and expertise-heavy multi-omics methods. An alternative approach, which captures the goal of assessing many different aspects of an animal’s biology while being much cheaper, is a “biomarker panel” approach, in which a large number of different easy-to-measure features that are associated with poor health in old age are collected from the same individuals. These could then be used as input to a machine-learning model which has been trained to use them to predict biological age. This approach has many advantages, including potentially high reliability and low cost. However, it seems to require a fairly high level of interaction with the animal (at least in humans and lab animals, many of the markers used are often behavioral), making it difficult to use in wild contexts, and is probably quite species-specific. Both of these issues could be overcome to some extent through the choice of biomarkers used. ↩︎
Jacobson, J., et al. (2010). Biomarkers of aging in Drosophila. Aging Cell 9 (4): 466-477. ↩︎
Zeichner, S. L., et al. (1999). Rapid telomere shortening in children. Blood 93 (9): 2824-2830. ↩︎
Hollingsworth, J. W., Hashizume, A., & Jablon, S. (1965). Correlations between tests of aging in Hiroshima subjects—an attempt to define “physiologic age”. Yale J Biol Med 38 (1): 11-26. ↩︎
In the case of telomere attrition, juveniles may actually be more appropriate subjects than adults (M. Bateson, pers. comm.): the base rate of telomere attrition is much higher, which makes changes in the attrition rate due to welfare factors easier to detect. It is also much easier, quicker and cheaper to do longitudinal studies on juveniles, avoiding many of the interpretation issues associated with cross-sectional studies. ↩︎
Charlesworth, B. (2000). Fisher, Medawar, Hamilton and the evolution of aging. Genetics 156 (3): 927-931. ↩︎
Dańko, M. J., Kozłowski, J., & Schaible, R. (2015). Unraveling the non-senescence phenomenon in Hydra. Journal of Theoretical Biology 382: 137-149. ↩︎
Ruby, J. G., Smith, M., & Buffenstein, R. (2018). Naked mole-rat mortality rates defy Gompertzian laws by not increasing with age. eLife 7:e31157. ↩︎
Dawkins, M. S. (2017) Animal welfare with and without consciousness. Journal of Zoology 301: 1-10. ↩︎
Bateson, M. et al. (2019). Smoking does not accelerate leucocyte telomere attrition: a meta-analysis of 18 longitudinal cohorts. Royal Society Open Science 6: 190420. ↩︎