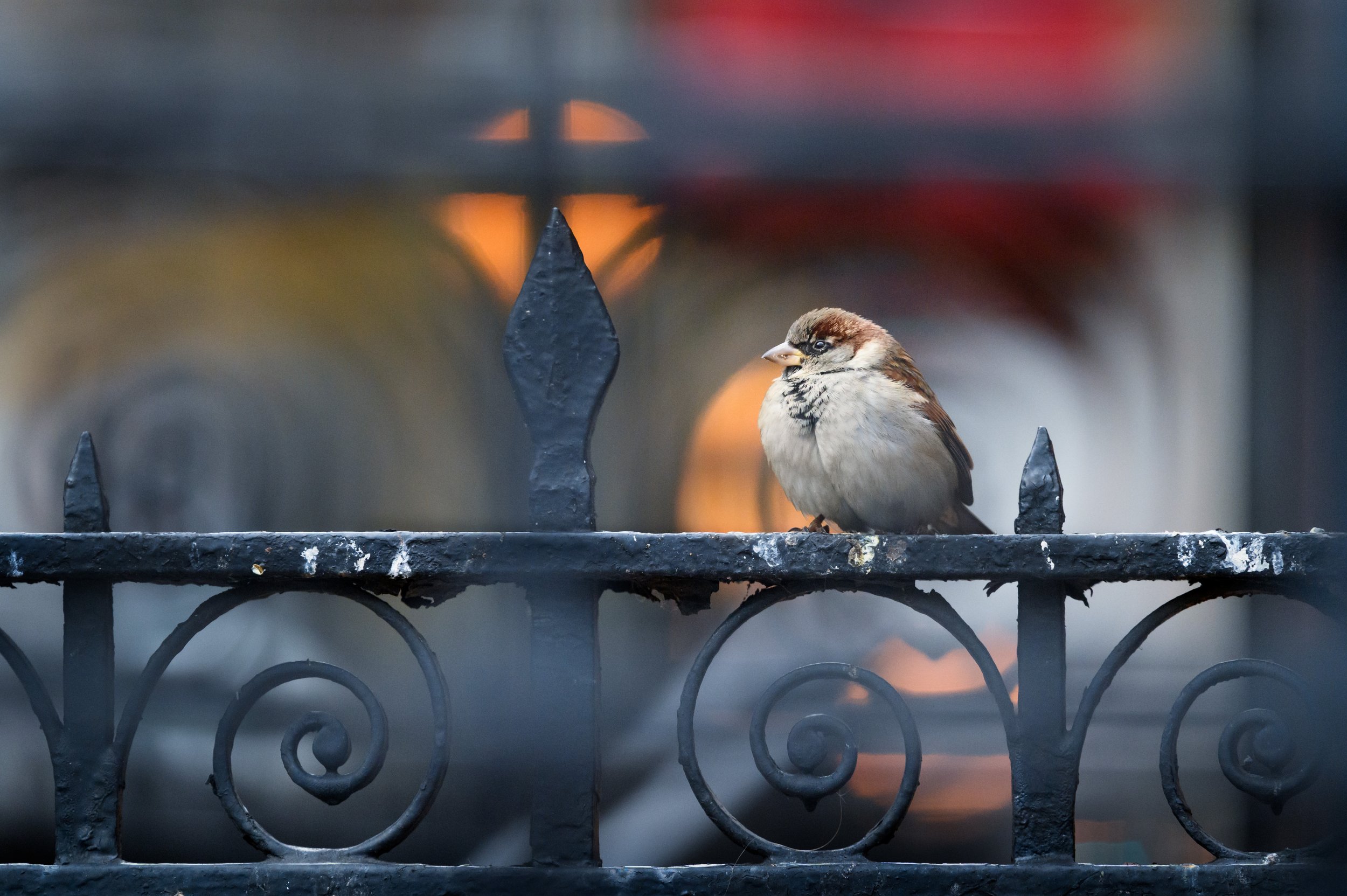
Library
Browse resources published by our research team.
In addition to full texts of our peer-reviewed articles, our library includes research digests that break down our peer-reviewed articles; in-depth reports that thoroughly examine a topic; commentaries that explain the significance of particular issues in wild animal welfare science; and short communications that briefly survey a field or topic.
Wild Animal Initiative adheres to Open Science TOP Guidelines. Read more here.
Improving wild animal welfare through contraception
Simon Eckerström Liedholm, Luke Hecht, Vittoria Elliott
Eckerström Liedholm, S., Hecht, L., & Elliott, V. (2024). Improving wild animal welfare through contraception. BioScience, https://doi.org/10.1093/biosci/biae071
Authored by Wild Animal Initiative’s Researcher Simon Eckerström Liedholm, Grants Manager & Researcher Luke Hecht, and Science Director Vittoria Elliott, this paper was published September 11, 2024, in BioScience.
Abstract
To date, research on the welfare impacts of wildlife contraceptives has mostly been focused on the potential harms of contraceptives. However, there are compelling theoretical reasons to expect direct and indirect welfare benefits of wildlife contraceptives. These positive welfare effects would be experienced by more than just the treated individuals, because per capita resource availability will increase with decreasing numbers of individuals sharing a resource. In the present article, we discuss the potential for wildlife contraceptives to alleviate resource competition and their associated negative welfare effects at different scales. These effects are expected to vary across contexts and would presumably be stronger when wildlife contraceptives are used with the explicit purpose of improving wild animal welfare. The potential for considerable welfare gains for wildlife through the targeted use of contraceptives highlights the importance of both species-specific studies on the welfare benefits of wildlife contraceptives and further research on the links between population dynamics and wild animal welfare.
Wildlife contraception and welfare
Simon Eckerström Liedholm discusses why contraception is a promising tool for improving wild animal welfare, and what further research is most urgently needed.
Contraception as a promising tool for welfare improvements
When resources are scarce, wild animals sometimes die of starvation (Gordon et al. 1988), dehydration, or exposure due to a lack of suitable shelters (Sibly & Hone 2002, Hill et al. 2019), all of which cause significant suffering (Gregory 2008). In many species, the competition for resources is especially strong among juvenile animals (Sol et al. 1998, Ward et al. 2006). Naively, making more resources available seems like it would solve the resource scarcity problem, but increasing the amount of available resources will often lead to higher fertility rates and juvenile survival rates, which eventually leads to larger populations instead (Prevedello et al. 2013, Ruffino et al. 2014), such that the competition for resources does not decrease in the long term.
One simple approach to find out how many animals are negatively affected by resource scarcity is to measure the fraction of deaths that are directly attributed to starvation. However, such an approach plausibly underestimates the pervasiveness of resource scarcity, due to interactions among different types of causes of death. For example, predation appears to be the most common cause of death for several taxa and life stages (Hill et al. 2019, Collins & Kays 2011), but whilst it is the proximate or final cause when it happens, it may not always be the ultimate cause — the underlying reason the lethal event occurred. For example, an animal that is starving may be prone to taking risks (Anholt & Werner 1995), or too weak to run away, which causes them to be more exposed to predators, making them more likely to be eaten. Therefore, even for some proximate causes of death that do not seem to be related to resource scarcity, there could be an underlying scarcity-related risk factor. In that case, a reduction of one cause of mortality (e.g. predation) might be partially or fully ‘compensated’ by the increase of another cause of mortality (e.g. starvation) such that life expectancy doesn’t change, known as compensatory mortality (Bergman et al. 2015). If everyone who is starving is eaten by a predator just before they would otherwise die of starvation, it would superficially appear like starvation wasn’t a problem at all, if we base our judgment solely on the proximate cause of death (predation in this case). Nevertheless, resource scarcity does appear to be a fairly common phenomenon (Prevedello et al. 2013), and irrespective of the ultimate cause of death, living a life of near starvation is a negative experience and of welfare concern. However, since resource limitations often are restraining population sizes (Prevedello et al. 2013), many populations will simply grow in response to increased resource availability, resulting in a larger population with similar levels of starvation.
Contraception provides one mechanism by which resource scarcity could be reduced, without inducing a corresponding increase in population size (Hecht 2021). Moreover, contraception may improve individual well-being through the alleviation of resource scarcity in other ways as well. For example, contraception could improve the welfare of the offspring by reducing sibling competition for resources (Mendl 1988, Andersen et al. 2011, Hudson et al. 2011). It could also reduce the burden of gestation and childrearing for some parents by allowing them to save energy and invest more in maintaining body condition (Kirkwood 1977, Kirkwood & Rose 1991, Lemaître et al. 2015). The cost of parental care, and thus benefits of reducing it, are dependent on the species and sex of the individual (Goldberg et al. 2020, Santos & Nakagawa 2012), which will cause additional variation in the net welfare effect of contraception.
There are several studies in which the effects of contraceptives on proxies for welfare have been investigated in wild animals (see Supplementary Table 2 in the review by Gray & Cameron 2010), and they seem to show both negative and positive welfare effects. The negative effects that were detected for some species/methods are related to maladaptive social behavior and inflammation at injection sites, among other things (Gray & Cameron 2010). The plausibly positive effects that have been found are related to survival and body weight, which are the types of benefits that we would predict given the model outlined in the previous paragraph. Several of the studies on survival detected increases (see for instance: Twigg et al. 2001, Turner & Kirkpatrick 2002, Williams et al. 2007), but one of them found no effect (Saunders et al. 2002), and two of them observed mixed results (Ransom et al. 2013 and Bromley & Gese 2001). Many studies have looked for changes in body weight, and several of them have found increases in treated females (see Table S2 in Gray & Cameron 2010). Such increases in body weight could serve as a proxy for welfare through improved nutritional status, but it could also be a reflection of changes in satiety and the foraging risk-reward trade off, where the ultimate effect on welfare is less clear. Many studies have had very small sample sizes, lacked control groups, and focused on a narrow set of welfare relevant traits, so it is hard to draw any strong conclusions. However, it seems very likely that the type, target and delivery mechanism of contraceptives have contributed to the observed differences in welfare-related outcomes among studies.
It is important to note though, that wildlife contraceptives haven’t been developed or applied with the goal of improving wild animal welfare by alleviating resource scarcity. As an analogy: even though a squirt gun is ineffective at putting out large fires — it was never intended for that use — water delivered in the right way is nonetheless very useful when there is a fire. Similarly, it is perhaps no surprise that the currently available evidence on the welfare effects is inconclusive, if no one has intentionally tried to improve wild animal welfare with it. It is the compelling theoretical potential that wildlife contraception offers for significant welfare improvement for large numbers of individuals that makes us excited to see further research in this area.
A very brief history of wildlife contraception research
As of 2011 (Kirkpatrick et al. 2011), wildlife contraception had been developed and successfully tested in 85 species of animals (mostly mammals), and work on different forms of wildlife contraception have continued since then (Asa & Moresco 2019). There are several different forms of contraceptives available for wild animals. Vaccine-based contraceptives (so-called immunocontraceptives) like the porcine zona pellucida (PZP) vaccine and the gonadotropin-releasing hormone (GnRH) vaccine were developed for feral horses and other wild mammals in the 1980s, and are still being used today (Asa & Moresco 2019). Steroid hormonal contraception methods have also been investigated, although high costs, toxicity, and problems with delivery (Kirkpatrick et al. 2011) have caused these methods to mostly be abandoned. Surgical sterilization has also been used to reduce fertility in wild populations of animals (Denicola & Denicola 2021), but because of the invasiveness of the procedure, it is likely more detrimental to animal welfare than other methods (Hampton 2017). It is also oftentimes more expensive per treated animal (Kirkpatrick et al. 2011). More recently, contraceptives have been developed for birds (Nicarbazin), such as pigeons and geese, and 4-vinylcyclohexene diepoxide (VCD) developed for rats (See box). These contraceptives prevent fertilization (in the case of Nicarbazin) or development of germ cells (in the case of VCD), and have only recently become available as commercial products. Products like these open up the potential for large-scale application of wildlife contraceptives, given the abundance of pigeons and rats in the world, and the potential for use in other related species.
Future research
Although scientists have conducted wildlife contraception research for decades, the focus of this research has largely not been on the welfare impacts of contraception, most likely because the primary goal has been to reduce population sizes of pest or managed species, rather than to improve welfare. Consequently, more research is needed to address the many unanswered questions regarding the possible connection between contraception and welfare. Examples of such questions include: can we expect better or worse welfare outcomes in certain taxa? If there is strong interspecific competition, will contraception lead to compensatory population growth and resource use by competitor species, eliminating the potential positive effects?
In addition to ecological considerations of contraceptive use, each of the methods requires additional scrutiny. How do different delivery methods, such as oral baits, injections and surgical sterilizations differ in their welfare effects? What are the welfare related side-effects, and in what contexts do they outweigh the possible positive welfare benefits? What are the long-term welfare effects to the treated animals? What are the indirect effects from changes in population sizes on the welfare of individuals belonging to other species?
Finally, the inter-individual dynamics of different animal populations generate additional research questions. For example, do the effects of contraception on welfare differ between solitary and social species? One reason we might expect different welfare outcomes in social and solitary species is that for social species, fewer offspring could affect the collective gathering of resources or protection from outside threats. A smaller group could be suboptimal relative to a larger social group, and contraception could therefore reduce the group size below its optimum and have negative welfare implications. Conversely, individuals of solitary species might mostly experience competitive interactions with conspecifics, where a smaller population size would be more likely to have positive welfare effects through reduced competition.
We outline a few potential projects that could help answer some of the questions regarding the welfare aspects of wildlife contraception:
Project idea 1: Empirically test the welfare effects of a contraceptive agent, with a focus on both the direct and indirect effects on individuals within the population. See an example for such a project on pigeons. Another target could be rodents (using, for example, the product ContraPest) or other species that have high fertility (with potential to improve the welfare of many individuals).
Project idea 2: Empirically or theoretically test whether and how sociality interacts with the potential welfare effects of contraception.
Project idea 3: Empirically test whether different delivery methods have different average welfare outcomes, and whether it is possible to predict which method will suit different types of species.
Project idea 4: Empirically explore the factors that determine perceptions of wildlife contraceptives, and determine public support for making improvements beyond the ‘natural’ welfare-level as opposed to just minimizing harms.
For all of the possible projects mentioned above, studying both adults and juveniles would be highly valuable when possible, given that we expect somewhat different mechanisms to mediate the welfare effects in adults compared to the welfare effects on juveniles. For instance, we would expect any reduction in sibling competition to primarily affect juvenile welfare.
Box 1
When looking for ways to test hypotheses about the welfare effects of contraceptives on wild animals, we want to look for projects where there is a potential for improvement to a lot of individuals. Rats (Rattus spp.) seem highly promising in that regard, given that they have high fertility rates. The reproductive strategy of rats results in a large number of ‘excess’ individuals who will most likely die before they reproduce. By reducing competition, contraception might increase the fraction of juveniles surviving to reproductive maturity, even if there is no change in the size of the adult population. Humans are also already exploring contraceptive interventions for them. ContraPest (developed by SenesTech) is a type of contraceptive that can be used for rats and other rodents in the United States. Testing the welfare effects of ContraPest on rodents, or indeed trying to further cut costs or develop additional types of contraceptives for rodents, seem like very promising avenues for further research.
Pigeons (Columba livia) are another promising target species for studying the welfare effects of wildlife contraceptives, as they are also very common, and there is a commercially available contraceptive for them (OvoControl, produced by Innolytics). The fertility rate of pigeons is lower than the fertility rate of rats, which therefore likely affects the potential for juvenile welfare improvement. Nevertheless, the available contraceptive for pigeons appears to be somewhat cheaper than for rats, as is indicated by my rough cost estimate for applying OvoControl and ContraPest to populations of pigeons and rats, respectively. In addition, public support for pigeon welfare (and other bird species) improvements is likely to be greater than for rats (Jaeger & Wilks 2021), so studying the welfare effects of OvoControl might have greater potential for widespread implementation. Hence, studying the welfare effects of pigeon contraception might be the most promising option, in order to eventually achieve welfare improvements for wild animals through contraception.
While currently available data does not clearly demonstrate welfare improvements from contraceptive applications, there are good theoretical reasons to expect that contraception could be extremely effective under the right conditions. Therefore, if we explicitly try to use contraception to improve wild animal welfare, it has the potential to improve the living conditions of many wild animals, especially in populations where resource scarcity is pervasive. Wildlife contraception holds great potential to improve wild animal welfare, and research to address the key theoretical and empirical questions should be a priority.
Improving pest management for wild insect welfare
Hollis Howe
Why should you care about insect welfare? Hollis Howe summarizes the literature on invertebrate sentience, estimates the number of insects affected by agricultural insecticide use, and describes the effects of common insecticidal compounds and other pest control methods.
Why insecticides?
Every year, approximately 100 million acres of American farmland are treated with lethal insecticides, killing or harming an estimated 3.5 quadrillion insects—according to our new report.
It’s currently uncertain whether insects can feel pain at all. But the sheer number killed by insecticides—and the potentially excruciating nature of those deaths—means that making pesticides more humane has a good chance of preventing large amounts of wild animal suffering.
Pesticide reform also has the advantage of being relatively easy to implement responsibly.
One of the biggest challenges to most wild animal welfare interventions is figuring out the net effect on all the species in the community. This is especially true when population sizes change. For example, falling wolf numbers might lead to rising coyote numbers, leading to falling fox numbers, leading to rising mouse numbers, leading to rising tick numbers—or they might not.
If we can replace one insecticide with another that kills the same number of insects less painfully, we can reduce suffering without changing the total insect population. That significantly narrows the range of possible unintended consequences.
The goal of this report is to lay a foundation for future projects to improve wild insect welfare by promoting more humane insect pest management practices.
Key takeaways
The evidence supporting insect sentience is sufficient to argue that, in combination with the large number of insects, we should afford some consideration to insect welfare.
Agricultural pest insect management practices may be a particularly tractable avenue for improving the expected welfare of a large number of insects.
At this point, key unknowns make it difficult to recommend a particular insecticide or non-insecticidal pest control method as more humane. However, nerve and muscle agents (such as organophosphates and carbamates, or pyrethroids and pyrethrins) are faster-acting than insecticides with other modes of action (such as insect growth regulators, or Bt toxins).
Non-insecticidal methods of pest control may be gaining popularity and should be considered when developing more humane pest insect management practices. The net welfare impact of non-lethal methods is dependent on ecological factors and the specific organisms involved.
Next steps
In addition to the report itself, I developed two tools to facilitate further research.
The first is a database of insecticidal compounds, their modes of action, and their insecticidal mechanisms. This database is under development and may be expanded to include pests targeted, brand names, and chemical fact sheets where available.
I have also developed a rough impact estimate table outlining a method for using a pest control literature review to calculate the minimum number of insects affected by U.S. agricultural insecticide use.
As part of our upcoming strategic planning and inter-organization coordination process, Wild Animal Initiative will be evaluating the course of our future research on insect welfare. Currently, it looks like the most promising direction would be to fill in the gaps necessary to evaluate specific insecticide interventions:
What are the economic injury levels (EILs) for particular crop-pest pairs?
Can EILs be used to generate estimates of the number of insects affected by pest management?
On which insect species and crops are particular insecticides or pest management methods used?
In the process of creating this report, many more questions arose than were answered. Despite this, the report provides an overview of the insect welfare landscape and the potential avenues for further intervention research.
Long-term design considerations for wild animal welfare interventions
How persistent and reversible should our interventions be? Simon Eckerström Liedholm explores two important factors in assessing the merits of interventions to improve wild animal welfare.
Persistence and reversibility
When assessing the merits of different interventions to improve wild animal welfare, two prominent factors are (1) how persistent a certain intervention is and (2) how easy it is to reverse the effects given a bad outcome.
Reversibility is important because we can’t always predict upfront exactly how an intervention will turn out. If things go wrong, we want to be able to reverse the intervention to contain the damage. We anticipate having a better empirical understanding in the future, so to preserve option value, we probably want to delay persistent and hard-to-reverse interventions until we’re highly confident they’ll have positive effects.
Persistence, or the duration of the effect in expectation, is important because it will affect how much effort we would have to put into improving wild animal welfare by a certain amount. If the effects are very persistent, we only have to initiate the process once, then we can reap the benefits over a long period of time.
However, the benefits of higher cost-effectiveness of persistent interventions seem to be strongly outweighed by other factors in most cases. Firstly, most highly persistent interventions are very hard to reverse, which means we have to give up a lot of option value. Secondly, trying to implement highly persistent and hard-to-reverse interventions could stir up a lot of animosity, which would be damaging for the long-term stability of the wild animal welfare project.
Thus, it seems like if we have to choose between an intervention with low persistence but high reversibility and an intervention with high persistence but low reversibility, we should choose the former rather than the latter.
Striking a balance
There might be a way, however, to increase persistence in some scenarios without paying with reduced reversibility. More precisely: if we could find interventions that are persistent in the face of natural processes, while at the same time being highly reversible by humans, we could get some of the benefits of persistence, while avoiding the negative consequences of implementing irreversible interventions.
With continued work on their efficacy and safety, gene drives might possess these characteristics in the future. A gene drive is a genetic modification that makes itself more likely to be inherited. Gene drives can be persistent because they are able to modify the genome of every individual in a population or species. Gene drives can also, under some circumstances, be reversible. A secondary gene drive can target the primary gene drive, halting its spread and removing the changes it made. However, it is important to note that the safety and reversibility of gene drives is critically dependent on how much effort is put into making them safe and reversible, which is one reason why the development of policies regulating gene drives will be of high importance.
Despite gene drives being an important exception, most actions will probably be either non-persistent and reversible, or persistent and non-reversible. In my report, I explore three examples in the second category: extinctions, climate change, and energy efficiency improvements in plants. Even though all of these examples have somewhat unclear effects on wild animal welfare, they should probably be avoided due to the fact that they seem very hard to reverse, and will persist for a long time.
Cover photo: Adapted from “Loggerhead sea turtle hatchlings crawl to sea,” © Blair Witherington, Creative Commons
Optimal population density: trading off the quality and quantity of welfare
Luke Hecht explains how, in some cases, reducing wild animals’ population densities by relatively small amounts might facilitate large increases in their welfare.
Introduction
Largely as a result of human development, the sizes of many wild animal populations have been falling.1 Much of this decline has resulted not from direct killing of wild animals by humans, but indirectly by fragmenting their habitats and cutting animals off from the resources that sustained their historical population sizes.2,3 This has immediate consequences for the welfare of individuals, who may face starvation, competition for resources, or conflict with humans as they try to access resources in their former habitat (e.g. urban pigeons4; fur seal5; black bear6). According to many ethical views, it is better to have more happy animals than fewer equally happy animals; but as a population grows, so does the number of individuals who may be adversely affected by further growth, requiring us to think carefully about trade-offs between the quantity and quality of welfare. A thorough understanding of individual welfare levels in a given population and how they are affected by changes in population density could eventually enable us to estimate the population size which would maximize the welfare experienced in that population.
Population modeling and density-dependence
The density of a population is defined by the ratio between the number of individuals and the amount of habitat or a limiting resource available to them; if a population grows or its habitat declines, population density increases. The population size (N) at which a balance between birth rate and death rate is achieved—its maximum sustainable size—is known as the “carrying capacity” (K) under given environmental conditions (Box 1).
Box 1
A population’s growth rate per generation (r) is equal to the difference between the number of births (b) and the number of deaths (d): r = b - d. The growth rate before any effects of density are taken into account is denoted r0. To the extent that r0 exceeds zero, density-dependent effects must reduce age-specific survival or fecundity rates to achieve a stable population. The population size (N) at which r = 0 and the population remains stable long-term is known as the carrying capacity (K).
These parameters come together in the logistic growth equation, where any excess of births over deaths shrinks to zero as N approaches K: dn/dt = r0N(K - N)/(K).
While this equation captures how extremes of population density relate to population growth rates, it is certainly incomplete. For example, intermediate densities may have less consistent effects than the simple logistic function suggests, especially in populations facing strong density-independent stressors. Populations inhabiting an environment that changes much more rapidly than the time between their generations may never reach their carrying capacity at any given time, but may still encounter strong density effects during periods when N > K, as might occur during a drought or similar period of low productivity.
Death rates may rise and birth rates may fall as a result of stressors experienced by the animals in a population. These stressors can be density-dependent or density-independent. For example, competition for food can lead to starvation and limit the energy available for reproduction7, and crowding in early life can cause an animal to age prematurely8. Infectious diseases may also spread more rapidly in a dense population9.
Other causes of mortality are density-independent. In a river, water temperature or pollutants might kill fish at a rate that is independent of the number of fish in the river10. Indeed, density-independent factors can make an environment so hostile that the maximum stable population size is very low, and the surviving individuals face no meaningful competition for resources.
Whether a certain factor is density-dependent or density-independent can also vary according to the circumstances. For example, the direct threat posed to any individual by fierce winds and lightning during a storm is independent of density, but density-dependent mortality might follow in the wake of the storm if a limiting resource has been damaged. Population density within a certain range can also have positive effects for individual welfare by providing safety in numbers and more accessible social interactions. For example, having fellow newts around during their transition to maturity means that individuals of Triturus cristatus are less likely to undertake dangerous migrations to find mates11.
Welfare implications of population density
In a recent article, I proposed the concept of welfare expectancy as a way to link age-specific survival rates and welfare. Welfare expectancy is a sum of the lifetime welfare an individual born into a given population can expect to experience. Age-specific survival rates may also be correlated with age-specific welfare, suggesting that any density-related impairment of survival could greatly reduce welfare expectancy, especially when it affects juvenile animals.
A general expectation of declining welfare expectancy per individual as a population grows towards its carrying capacity raises the possibility that the population size which maximizes the total welfare of a population may be smaller than its maximum sustainable size. Whether this occurs depends on how many individuals the population would contain at its carrying capacity and how average welfare varies with population size (Box 2). The conditions are most likely to be met if the strongest negative effects of population density on welfare manifest at high densities (i.e., as N approaches K; Figure 1). This could occur under the following three conditions:
- if lower densities primarily limit reproduction while higher densities affect survival rates.
- if density-dependent mortality disproportionately affects older animals at lower densities but young animals at high densities; or
- if density-independent effects are nearly sufficient to stabilize the population’s growth rate, so any negative effects of density are only felt near the carrying capacity.
In general, the shape of average welfare expectancy with respect to density depends on the particular ecology and life history of the population in question, especially whether density preferentially affects fecundity versus survival rates or the survival rates of young versus old individuals. If increased density is mainly accommodated by reduced fecundity or late-life survival, for instance, then average welfare might not decline so steeply as a population grows. Theory and reviews of demographic models for a variety of large vertebrates suggest that juvenile survivorship is usually the first casualty of high population densities12,13,14, which is one set of conditions under which we might expect the welfare-optimal population density to be lower than the population’s carrying capacity. Part of the explanation for this pattern in large vertebrates is the competitive advantage older animals typically have over younger animals who are smaller and less experienced. However, it is not yet known how broadly these age dynamics apply among the much more numerous smaller-bodied, “fast-living” animals, where there are examples of adult advantage15 and disadvantage16. More research is needed into the expected shape and mechanisms of density-dependence among their populations.
Box 2
Let WN represent the average welfare expectancy at population size N and let K represent the population size at carrying capacity. Then, the welfare-optimal population size will be less than the carrying capacity if WK-1/WK > K/(K - 1).
The welfare expectancy per individual in a population depends on the life expectancy from birth and the welfare they typically experience over that lifespan. If average welfare is positive, average welfare expectancy will correlate with life expectancy. If an increase in population density disproportionately harms the youngest animals, this can greatly reduce life expectancy.
Implications for welfare interventions
If we are completely uncertain about the absolute welfare level of animals in a population, then it follows that we are completely uncertain about whether their lives are dominated by pleasure or suffering. If their lives contain more goodness on average, then increasing the population size would be expected to increase the total amount of welfare experienced within the population as a whole. On the other hand, if their lives are primarily unhappy , then adding more unhappy individuals through population growth would actually subtract from the population’s total welfare. From a standpoint of complete uncertainty, then, the expected value of population growth or decline is symmetrical17. Whether or not population growth increases or decreases total welfare of the population depends on the balance of the positive and negative experiences of the individuals added by that growth.
However, the existence of a density-dependent decline in average welfare would set up an asymmetry in favor of relatively smaller populations, since increasing population density would reduce the probability that average welfare remains net-positive, giving population growth a lower expected value than population reduction. Of course, this heuristic assumes complete ignorance of the population’s welfare characteristics, which is rarely the case.
On the other hand, if we knew the exact average welfare expectancy for all possible population sizes (WN), we could predict its optimal density and also define a ‘margin of error’ on either side within which total welfare expectancy would be expected to at least equal that obtained at carrying capacity (Figure 1). This could provide a wide target for interventions aiming to improve welfare by managing population density. Notably, the largest range and scope for improvement in total welfare over what would occur naturally is possible when the most aversive effects of population density only manifest at high densities. This suggests that a review of evidence on the shapes of density-dependence curves for various species could indicate how impactful managing around population density might be as a form of welfare intervention.
Figure 1. Left: Hypothetical curves depict how average (per-individual) welfare expectancy might decline with increasing population density, depending on whether the most aversive effects of density develop at low densities (“early” in population growth), at a constant rate (“continuous”), or only at high population densities (“late”). Right: Average welfare under each of these three scenarios is multiplied by population density to give total welfare. Total welfare below the level achieved at the maximum sustainable size (K) is shaded. Despite average welfare always being positive, the welfare-optimal population size (O) is less than the maximum sustainable population size under the “late” (O=0.75) and “continuous” (O=0.81) scenarios. Total welfare continues to increase up to K under the “early” model (O=1.00), where higher population densities have a smaller effect on average welfare.
Figure 2. Left: As the population grows from 0 to K average welfare declines from net-positive to net-negative (red shading). A population of wild animals might appear to have very poor welfare at their natural population density, yet have the potential for good welfare at lower population density. Right: In such a case, their total welfare would be maximized by sustaining a medium-sized population, with “Late” density effects increasing the population’s optimal size and potential welfare.
Population density could be managed (and any effects ameliorated) by reducing birth rates, culling individuals from the existing population, or increasing resource availability. Culling is currently the default method for managing animal densities, usually targeting those considered “pests.” Although welfare is occasionally considered, this approach is suboptimal because it involves inflicting suffering on some animals and cutting their lives short (even though culling methods may be less painful than natural causes of death18). Non-lethal reduction of population density could be achieved through fertility control, directly limiting the number of animals born without shortening the lives of extant individuals. A complementary approach is reducing the effective density of a population by increasing the quantity or quality of its habitat using the principles of restoration ecology. This could reduce density and improve welfare in the short-term without requiring any reduction in population size. However, without additional measures artificially limiting their growth rate, populations would simply return to their natural sizes and any associated harmful effects of density, so to be sustainable long-term this would still need to be paired with fertility control or another method. A combination of fertility control and habitat improvement could form the basis of a welfare-focused approach to wildlife management. Such an approach must also take into account non-target impacts on other species before being put into practice19.
Conclusions
It is currently technologically infeasible to make population management decisions that consider the needs of every individual wild animal. We therefore need to determine guiding principles to help us predict the likely results of various population management projects, even under uncertainty about the absolute level of welfare the relevant animals experience. Understanding the density-dependence of welfare in a given population provides one such principle. Furthermore, given sufficient information about absolute welfare levels, this approach could also be used to identify a window of effect sizes where an intervention is expected to increase total welfare relative to the natural long-term size of a population.
The effects of varying levels of density on survival and reproductive rates are exceedingly difficult to determine for any population, given the generational timescales involved and the fact that any population density other than K must by definition be unstable without outside intervention. As a result, precise estimates of density-dependence are rare in the literature, though there is empirical and theoretical support for certain generalizations. Direct evidence on wild animal welfare is also lacking, though we can make inferences from survival rates, body condition and behavior. Research in both of these areas could be high-impact, as an understanding of how welfare expectancy varies with population size would be crucial for planning many large-scale wild animal welfare interventions.
References
-
World Wildlife Federation. 2018. Living Planet Report - 2018: Aiming Higher. ↩
-
Andren, H. (1994). Effects of habitat fragmentation on birds and mammals in landscapes with different proportions of suitable habitat: a review. Oikos, 355-366. ↩
-
Ripple, W. J., Wolf, C., Newsome, T. M., Hoffmann, M., Wirsing, A. J., & McCauley, D. J. (2017). Extinction risk is most acute for the world’s largest and smallest vertebrates. Proceedings of the National Academy of Sciences, 114(40), 10678-10683. ↩
-
Senar, J. C., Montalvo, T., Pascual, J., & Peracho, V. (2017). Reducing the availability of food to control feral pigeons: changes in population size and composition. Pest management science, 73(2), 313-317. ↩
-
Doidge, D. W., Croxall, J. P., & Baker, J. R. (1984). Density‐dependent pup mortality in the Antarctic fur seal Arctocephalus gazellu at South Georgia. Journal of Zoology, 202(3), 449-460. ↩
-
Murphy, S. M., Augustine, B. C., Ulrey, W. A., Guthrie, J. M., Scheick, B. K., McCown, J. W., & Cox, J. J. (2017). Consequences of severe habitat fragmentation on density, genetics, and spatial capture-recapture analysis of a small bear population. PloS one, 12(7), e0181849. ↩
-
McMahon, C. R., Harcourt, R. G., Burton, H. R., Daniel, O., & Hindell, M. A. (2017). Seal mothers expend more on offspring under favourable conditions and less when resources are limited. Journal of Animal Ecology, 86(2), 359-370. ↩
-
Nettle, D., Monaghan, P., Gillespie, R., Brilot, B., Bedford, T., & Bateson, M. (2015). An experimental demonstration that early-life competitive disadvantage accelerates telomere loss. Proceedings of the Royal Society B: Biological Sciences, 282(1798), 20141610. ↩
-
Civitello, D. J., Allman, B. E., Morozumi, C., & Rohr, J. R. (2018). Assessing the direct and indirect effects of food provisioning and nutrient enrichment on wildlife infectious disease dynamics. Philosophical Transactions of the Royal Society B: Biological Sciences, 373(1745), 20170101. ↩
-
Boulenger, C., Acou, A., Gimenez, O., Charrier, F., Tremblay, J., & Feunteun, E. (2016). Factors determining survival of European eels in two unexploited sub‐populations. Freshwater Biology, 61(6), 947-962. ↩
-
Cayuela, H., Schmidt, B. R., Weinbach, A., Besnard, A., & Joly, P. (2019). Multiple density‐dependent processes shape the dynamics of a spatially structured amphibian population. Journal of Animal Ecology, 88(1), 164-177. ↩
-
Eberhardt, L. L. (1977). "Optimal" Management Policies for Marine Mammals. Wildlife Society Bulletin, 162-169. ↩
-
Gaillard, J. M., Festa-Bianchet, M., & Yoccoz, N. G. (1998). Population dynamics of large herbivores: variable recruitment with constant adult survival. Trends in Ecology & Evolution, 13(2), 58-63. ↩
-
Bergman, E. J., Doherty, P. F., White, G. C., & Holland, A. A. (2015). Density dependence in mule deer: a review of evidence. Wildlife Biology, 21(1), 18-30. ↩
-
Figler, M. H., Cheverton, H. M., & Blank, G. S. (1999). Shelter competition in juvenile red swamp crayfish (Procambarus clarkii): the influences of sex differences, relative size, and prior residence. Aquaculture, 178(1-2), 63-75. ↩
-
Fletcher, W. J. (1988). Intraspecific interactions between adults and juveniles of the subtidal limpet, Patelloida mufria. Oecologia, 75(2), 272-277. ↩
-
Briggs, R. A., "Normative Theories of Rational Choice: Expected Utility", The Stanford Encyclopedia of Philosophy (Fall 2019 Edition), Edward N. Zalta (ed.). ↩
-
Bradshaw, E. L., & Bateson, P. (2000). Welfare implications of culling red deer (Cervus elaphus). Animal Welfare, 9(1), 3-24. ↩
-
Clarke, M., & Ng, Y. K. (2006). Population dynamics and animal welfare: issues raised by the culling of kangaroos in Puckapunyal. Social Choice and Welfare, 27(2), 407-422. ↩
Age-specific survivorship frames the expected value of wild animal welfare
This research note is an extension of Luke Hecht’s paper, The importance of considering age when quantifying wild animals’ welfare, which was published in Biological Reviews in December 2021. Luke introduces “welfare expectancy” as a framework for summing up the different levels of well-being animals might experience over the course of their lives, helping to compare the welfare consequences of interventions and natural pressures that may disproportionately affect animals of particular ages.
This project is a continuation of work that began at Animal Ethics, which is covered here. We thank them for their collaboration.
As part of an ongoing project to understand the welfare of wild animals, I analyzed age-specific survivorship as it relates to welfare, introducing a new concept for understanding the lives of wild animals: welfare expectancy. Welfare expectancy can serve as a framework for weighing up the different levels of well-being animals might experience over the course of their lives, helping to model the welfare consequences of interventions and natural pressures, such as predation, that may disproportionately affect animals of particular ages.
Wild animals’ lives are extraordinarily diverse. Individuals of different species occupy different habitats, consume different resources, and engage in different behaviors. Even within species, their fortunes vary depending on their circumstances of birth and exposure to chance events which lead to differential survival, mating success, and welfare.
Organisms must make trade-offs between their own survival and reproduction, and the survival of their offspring. These trade-offs evolve to maximize lifetime reproductive output, which defines an individual’s fitness. However, it is crucial to recognize that fitness and welfare are not the same. For example, a strategy which maximizes the average fitness of offspring could lead to reproductive success in adulthood for a few, but short lives for most.
In order to identify safe and tractable ways to improve wild animals' lives, welfare biology needs to identify the major threats to their welfare. In the face of the diversity of individual experiences wild animals may have, we need a way to tally them up in order to assess the overall welfare of a population. Expected value does this by taking the sum of the value of each outcome multiplied by its probability. For example, to calculate the life expectancy of a population (i.e. the expected value of lifespan), one would multiply the proportion of individuals who die at a certain age by the number of years they lived and sum this across all possible lifespans.
In my forthcoming paper (previous version), I introduce a similar concept of “welfare expectancy” to formalize the relationship between age-specific survivorship and lifetime welfare. Consider a species for which welfare is poor in early life, but high in adulthood. If the probability of surviving early life is high, then the lifetime expected value of welfare for an individual born into that population may be high, because most individuals are going to have a chance to live out their best years in adulthood. If the probability of surviving early life is low, then most individuals will only live to experience the juvenile period of poor welfare. Conversely, in a species where welfare is higher in early life than in adulthood (e.g. due to good parental care), the net welfare of even a short-lived animal could be relatively high.
We are profoundly uncertain about whether most animals’ lives are dominated by pleasure or suffering, or even how to go about weighing these up. Therefore, it may be prudent to concentrate on a measure of “relative welfare expectancy” (RWE), representing the normalized welfare expectancy of a population divided by its life expectancy. For a fixed life expectancy, the highest welfare expectancy is achieved by maximizing the proportion of animals living to experience the best years of life while minimizing the proportion experiencing the worst years, as illustrated in Figure 1.
Figure 1: Assuming a fixed maximum lifespan, relative welfare expectancy is maximized when the majority of individuals live through ages characterized by above-average welfare. For example, in the “bad” case, the highest-welfare periods come late in life; most individuals in such a population only experience the poorer-welfare early ages.
As part of an investigation of age-specific mortality jointly supported by Animal Ethics and Wild Animal Initiative, I analyzed published demographic models for 152 populations of 88 species to survey patterns of age-specific mortality and used them to illustrate the implications of age-specific welfare. The vast majority of animals live very short lives; not only in absolute terms, but also relative to the longest-lived members of their species. In fact, of the populations analyzed, average lifespans were on average 16% of a species’ maximum lifespan, with only 5% of populations having life expectancies >33% of their maximum (Figure 2). Importantly, this represents an average across populations, not across individuals. Because short-lived species tend to be more populous, lives in nature are likely to be cut short far more often than these numbers suggest. Depending on how welfare varies with age in their respective species, especially short-lived individuals will be missing out on a great deal of positive and/or negative experience.
Figure 2: Histogram of life expectancy as a proportion of the species’ theoretical lifespan. Most animals live only a small fraction of their potential lives, even in species where the theoretical lifespan is already relatively short.
Confident application of the welfare expectancy concept will require empirical data on values of age-specific welfare, which are currently scarce for wild animal populations. A plausible working hypothesis, however, is that the average day-to-day welfare experienced by an animal of a given age is proportional to their probability of surviving that period of life. The justification for this is that the same factors which lead to mortality (e.g. disease, vulnerability to predators, competition for food) have been shown to lead to chronic stress and poor physical condition.
Although we do not know where to draw the line between net-positive and net-negative welfare for any species, under this hypothesis the probability that welfare over a given period is net positive would also be proportional to the survival probability. For example, a year in which an animal has a 60% chance of surviving might be twice as likely to be net-positive as a year which they have a 30% chance of surviving. In the absence of evidence, for the purposes of this report we decided to represent age-specific welfare exclusively as a positive number ranging from 0 to 1 (equal to the survival rate), for illustrative purposes only. Readers are free to adjust the welfare expectancy values given in Table A1 of the report according to where they feel the line between net-positive and net-negative welfare may lie for any particular species by subtracting the product of its life expectancy and the minimum annual survival rate they feel would correspond to net-positive welfare.
For an animal to have an enjoyable life on net, they must experience enough pleasure to compensate for the pain of their death. Cause of death, and therefore the duration and pain of an animal’s experience of dying, may also vary with age similarly to welfare. In a hypothetical species, juveniles might be most likely to starve while adults are most likely to be predated, with the relative probabilities of these and other mortality factors shifting over a lifetime. If the pain of death is a sufficiently strong factor to negate some of the positive welfare an animal might have experienced while alive, age-specific variation in the incidence of various manners of death and their severity would also be important to account for. (See Michael Plant and Brian Tomasik for contrasting views on this.)
At the individual level, welfare expectancy unites two distinct concepts: day-to-day quality of life and the quantity of welfare experienced over an individual’s lifetime. However, a similar quantity-quality distinction applies at the level of populations, with welfare expectancy addressing the quality side of the argument and quantity being determined by the population size. Ideally, a population should be managed in such a way that maximizes its total welfare expectancy. A predictive understanding of population ecology is therefore crucial for contextualizing information on wild animal welfare, as well as evaluating and prioritizing among interventions that differentially affect various age or stage groups. The welfare expectancy framework might serve as an early paradigm for welfare biology.